The first thing that comes to mind when people think of insects is probably not their tiny brains. However, scientists have been studying and taking advantage of the relatively simple structure of insect brains for years, particularly when it comes to protecting crops. The first recorded use of pesticides dates back to almost 4,500 years ago when ancient Sumerians dusted their food plants with sulfur to keep insects and mites from destroying their food [1]. In the early 20th century, scientists in Europe began to elucidate the mechanisms behind pest-controlling compounds and used them to invent even more effective insecticides [1]. Over the past century, several classes of chemicals have been used in agriculture, the vast majority of which target specific proteins and processes that can cause devastating disruptions to the insect’s nervous system. Remarkably, the proteins and processes that govern the insect’s neural network are often the same as those found in higher-functioning animals, including humans [2]. This raises many questions regarding the level of risk that today’s insecticides pose to non-target organisms and how we can maintain stable levels of food production while minimizing damage to the balance of surrounding ecosystems. Understanding exactly how these neurotoxic, insect-killing substances work has the power to put the risks of widespread insecticidal use into perspective and will allow the public to make informed decisions about the regulation of such potent materials.
One of the most well-known groups of insecticides belongs to a class of chemicals known as the organophosphates, which are phosphorus-containing organic molecules. They were introduced to the world as revolutionary pest-controlling compounds in the 1930s when German chemist Gerhard Schrader discovered the powerful insect-killing abilities of many organic compounds [3]. Organophosphates work by irreversibly binding to specific enzymes found on the cell membranes between insect neurons [2]. The gap where one neuron meets another is known as the synaptic cleft. It is within this cleft that neurotransmitters are released to send the signals necessary for the neuron-to-neuron communication governing nearly every neural process in living organisms [4]. The message sent from one neuron to another depends on the identity of the neurotransmitter being released and its successful binding to specific receptors on the other neuron. The more neurotransmitter in the cleft, the higher the chances of it binding to its correct receptor, and consequently the higher the chances of a signal being sent. In cases where some of the receptors are damaged, it is useful to increase the amount of neurotransmitter in the cleft in order to counteract the decreased signal conduction due to the deficient receptors. One way scientists have been able to increase the amount of neurotransmitter in the cleft is by inhibiting the enzymes responsible for breaking down neurotransmitters. Without enzymes to clear them from the cleft, the neurotransmitters maintain higher levels in the synapse, binding to receptors and sending their signals more often [4].
The first neurotransmitter to be discovered, acetylcholine (ACh), is one of the most ubiquitously present compounds in the nervous systems of all living species. The enzyme responsible for its degradation in the synaptic cleft, acetylcholinesterase (AChE), is commonly targeted for inhibition by insecticides [5]. Limiting the function of AChE leads to the accumulation of ACh in the synapse. This in turn increases ACh’s effect on many biological systems, most notably in neuromuscular junctions, the gap between motor neuron axon terminals and muscle cells. Under normal conditions, the brain releases ACh into the neuromuscular junction to trigger muscle contraction. Once ACh binds to its receptor, it triggers the opening of a series of ion channels in the muscle fiber. If the ACh is not rapidly broken down by AChE, the muscle cells become hyperstimulated and cannot relax [5]. Organophosphorus insecticides permanently bind to AChE, inhibiting the enzyme’s function so it is unable to break down ACh in the synaptic cleft, leading to hyperstimulation of the muscle fibers. As a result, the muscles are unable to relax, leading to convulsions and eventually death. After observing this powerful effect in insecticides, German scientists realized the phenomenon could be extended to humans, which led to the discovery of the incredibly lethal nerve agents developed during World War II [3]. Today, AChE inhibitors, many in the organophosphorus class, are the most widely used pesticides. However, due to the similarity in neural function between insects and the majority of other organisms and the commonality of ACh as a vital neurotransmitter, the potential for these insecticides to cause harm to non-insect species remains a concerning topic, especially for those who work in the agricultural industry and experience high exposure to these compounds [2].
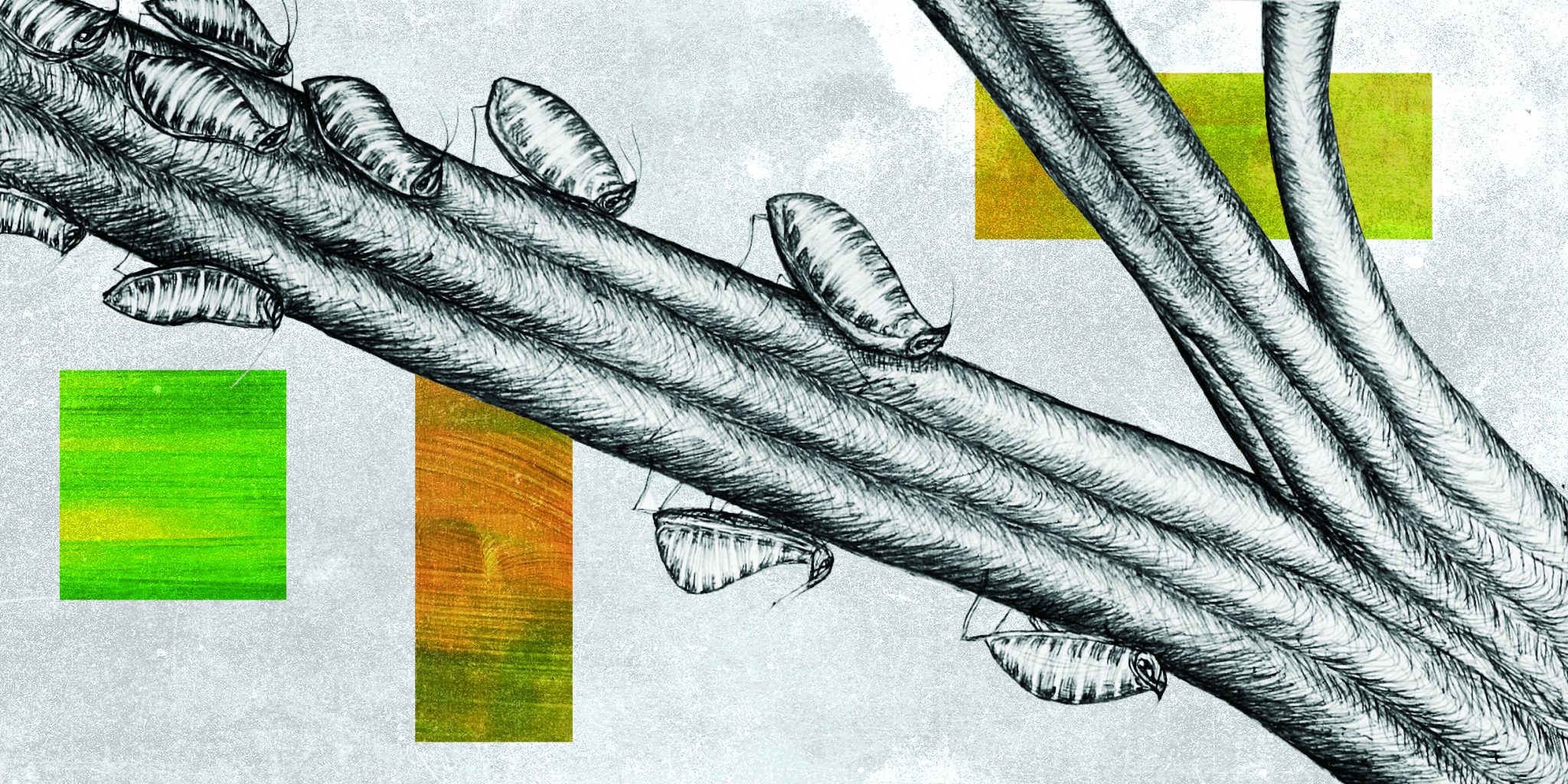
While stopping the enzymes responsible for neurotransmitter degradation is one extremely common way to increase neural signals, synthetically modifying the neurotransmitter receptors themselves is another equally effective and widespread insecticide mechanism in use today. The nicotinic acetylcholine receptor (nAChR) is a protein also located on cell membranes in the synaptic cleft that is essential for the regulation of muscle contraction [6]. These receptors are able to bind to ACh like normal ACh receptors but are more commonly targeted by insecticides due to nAChR’s faster activation rates [6]. Insecticides can target these receptors through either antagonistic (preventing normal function) or agonistic (activating normal function) means. If an insect’s nAChRs are artificially stimulated, the insect experiences the same effects as when its AChE enzymes are inhibited: spasms, hyperactivity, and eventually a painful, convulsive death [6]. If the insecticide functions as a nAChR antagonist, however, the target insect will not experience the normal effects of ACh and is paralyzed as a result. Many types of nAChR antagonists are actually used in anesthesia in human medical practice today because they allow doctors to temporarily paralyze muscles during surgical procedures. Both types of insecticides are also widely used in agriculture and pose similar risks to non-target species like worms, birds, and amphibians due to their mechanisms acting on such ubiquitous physiological characteristics [2].
Many neurotransmitters can bind to a variety of receptors, often opening a specific kind of ion channel that controls the resultant effect on the organism. Gamma-aminobutyric acid (GABA) is a neurotransmitter that functions similarly to ACh with one important difference: when it binds to its receptor, it causes an inhibitory rather than stimulating effect [7]. Within the complex neural network, there can be thousands of neuron-to-neuron connections at any given location, and one way the brain regulates multiple signals is through the use of interneurons that counteract other signals. In humans, this is responsible for the concept of opposing muscle groups; the message to contract is sent to both muscle groups, but interneurons negate the signals sent to one, allowing one muscle group to relax as the opposing one contracts. GABA is the main neurotransmitter released to cause this signal negation [7]. Insecticides can also target GABA receptors (GABA-Rs) through antagonistic or agonistic means. If the insecticide functions as a GABA-R antagonist, the targeted insect loses the ability to reduce signals it normally modulates and experiences hyperactivity and convulsive effects, similar to the excess ACh cases. If the insecticide is a GABA-R agonist, it works by attaching to and artificially stimulating GABA receptors, resulting in the cancellation of signals, which causes paralysis and eventually death [8].
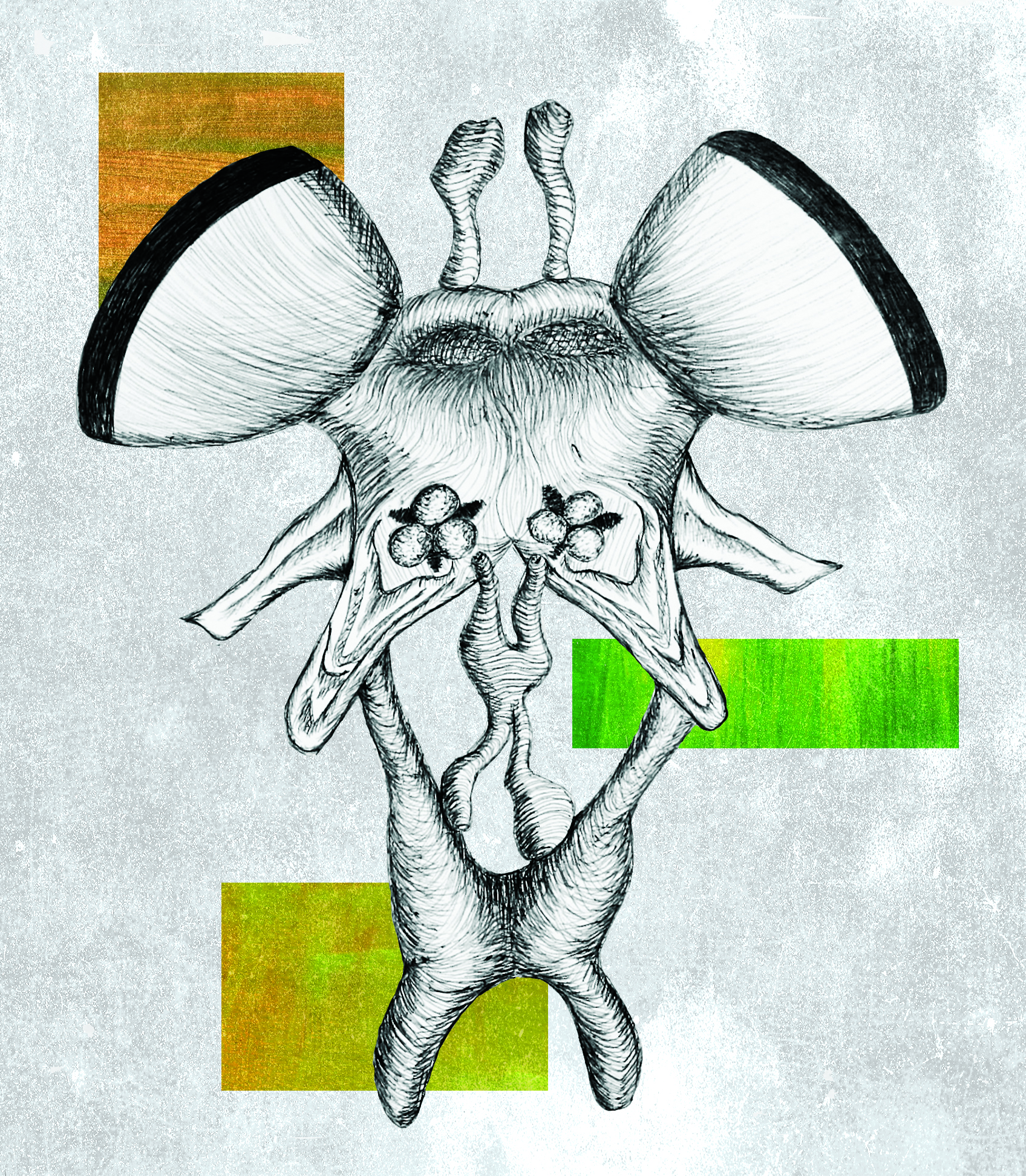
The mechanisms that make most insecticides so effective are also what make them so dangerous. When a chemical compound acts on structures found across a vast range of species, it can affect each of those species under the right conditions unless they evolve different structures, which seems to be the case with some GABA and nAChR proteins [2]. The susceptibility of non-target organisms to these highly effective insecticide classes can vary widely depending on minute differences in their receptor protein structures. Differences like these are responsible for the same chemical being harmless to a bee and simultaneously lethal to all bird species. But underneath the variations in their proteins, how these receptors actually control signals—through the opening of various ion channels—is the same [4].
Nearly every single physiological process is driven by gradients of charged ions. A delicate balance is maintained between the chemical concentrations and charges found on the inside and outside of neural cell membranes. Just like a charge flows along a wire to power a lightbulb, charges flowing along neurons send signals in the nervous systems of all living organisms. The flow of these charges is tightly controlled by ion channels along the neural membranes, and it is the receptor proteins previously discussed that control when these ion channels open and close [4]. By designing chemical compounds that change the functioning of the receptors, insecticides are able to indirectly affect ion channel modulation.
However, certain chemicals can also target the ion channels directly, leading to widespread toxic effects. This was exactly what Swiss chemist Paul Muller discovered in 1939 when he revealed that an organic compound, dichlorodiphenyltrichloroethane (DDT), was incredibly successful at wiping out a huge range of insect pests that were ravaging crops and spreading disease at the time [9]. DDT, and insecticides like it, target sodium channels in neural cell membranes that normally open when the cell receives a sufficient amount of stimulus to send a signal. The opening of the sodium channels is the first step in generating the ion flow necessary for the neuron to propagate a signal. After positively charged sodium ions flow into the cell for a certain amount of time, other ion channels open to restore the balance of charge and concentration within the neuron, and the sodium channels slowly close. If the sodium channels are forced to stay open, the balance cannot be restored and the cells are unable to send their signals, resulting in the organism’s paralysis. Likewise, if the channels cannot open in the first place, the signal transduction also ceases, and the organism cannot send signals to regulate any of its biological processes, leading to death [10]. The danger of using such a widely applicable, potent compound was quickly realized by governments around the world after they observed environmental residues and their adverse effects on human health and surrounding ecosystems. The use of DDT was banned worldwide under the Stockholm Convention in 2001 [11]. By proving to be an effective solution to important social issues like the preservation of crop production or the elimination of malaria-causing mosquitoes in Africa, while simultaneously being one of the most threatening insecticides on the market today, DDT’s neurotoxic effects bring awareness to the central paradox of pesticide use that is a main topic of agricultural debates today [12].
Humans have been able to develop efficient, cheap, and devastatingly effective insecticides by targeting the main components of the insect nervous system, and these chemicals have proved to be an invaluable asset to agriculture in the past. The caveat is that their neural network targets are not unique to insect systems but are in fact common to the overwhelming majority of species, humans included. As public focus increasingly shifts to less environmentally harmful forms of agricultural production, more attention is being placed on natural symbiotic relationships as an alternative to the current insecticides that pose risks well worth considering as we move forward.
References
- Taylor, E. L., Holley, A. G., & Kirk, M. (2007). Pesticide: A Brief Look at the History. Southern Regional Extension Forestry, 1-7. doi:SREF-FM-010.
- Sanchez-Bayo, F. (2012). Insecticides Mode of Action in Relation to Their Toxicity to Non-Target Organisms. Journal of Environmental & Analytical Toxicology, 4(2), 1-7. doi:10.4172/2161-0525.s4-002.
- Ganesan, K., Raza, S. K., & Vijayaraghavan, R. (2010). Chemical Warfare Agents. Journal of Pharmacy and BioAllied Sciences, 2(3), 166-178. doi:10.4103/0975-7406.68498
- Ferreira, A., & Paganoni, S. (2002). The Formation of Synapses in the Central Nervous System. Molecular Neurobiology, 26(1), 069-080. doi:10.1385/mn:26:1:069.
- Colovic, M. B., Krstic, D. Z., Lazarevic-Pasti, T. D., Bondzic, A. M., & Vasic, V. M. (2013). Acetylcholinesterase Inhibitors: Pharmacology and Toxicology. Current Neuropharmacology, 11(3), 315-335. doi:10.2174/1570159×11311030006.
- Shao, X., Xia, S., Durkin, K. A., & Casida, J. E. (2013). Insect nicotinic receptor interactions in vivo with neonicotinoid, organophosphorus, and methylcarbamate insecticides and a synergist. Proceedings of the National Academy of Sciences, 110(43), 17273-17277. doi:10.1073/pnas.1316369110.
- Mizuta, K., Xu, D., Pan, Y., Comas, G., Sonett, J. R., Zhang, Y., . . . Emala, C. W. (2008). GABAA receptors are expressed and facilitate relaxation in airway smooth muscle. American Journal of Physiology-Lung Cellular and Molecular Physiology, 294(6). doi:10.1152/ajplung.00287.2007.
- Bloomquist, J. R. (2003). Chloride channels as tools for developing selective insecticides. Archives of Insect Biochemistry and Physiology, 54(4), 145-156. doi:10.1002/arch.10112
- Fischer, G. (n.d.). The Nobel Prize in Physiology or Medicine 1948. Retrieved from https://www.nobelprize.org/prizes/medicine/1948/ceremony-speech/
- Vijverberg, H. P., Zalm, J. M., & Bercken, J. V. (1982). Similar mode of action of pyrethroids and DDT on sodium channel gating in myelinated nerves. Nature, 295(5850), 601-603. doi:10.1038/295601a0.
- Magulova, K., & Priceputu, A. (2016). Global monitoring plan for persistent organic pollutants (POPs) under the Stockholm Convention: Triggering, streamlining and catalyzing global POPs monitoring. Environmental Pollution, 217, 82-84. doi:10.1016/j.envpol.2016.01.022.
- Stolberg, S. G. (1999, August 29). DDT, Target of Global Ban, Finds Defenders in Experts on Malaria. The New York Times.