As we go through our daily routines, whether they be in a bustling coffee shop packed with sleep-deprived students or in the high-ceilinged library reading room, we are surrounded by the rustling pages of books, pings emitted by text messages, and emails full of words that we immediately consume. As we submerge ourselves into this plethora of words, we are perhaps blissfully unaware of the various connections and signals our brain is processing. Why is it that we find the word eloquent quite different than vivid? What makes us cry when we read about the tragic end of one of our favorite characters in a book? Research utilizing fMRI has shown evidence that when individuals are presented with words such as school or phrases such as leathery hands, our brains frequently associate these words with our own experiences in school or with our visual perception of leather [1]. These results suggest that not one but multiple parts of the brain, such as the left and right hemispheres and the cerebral cortex, work together to recall our memories of the smell of #2 pencils and homework we so often associate with school. It appears processing words and associating them with emotions is a much more complex and intertwined system than previously thought.
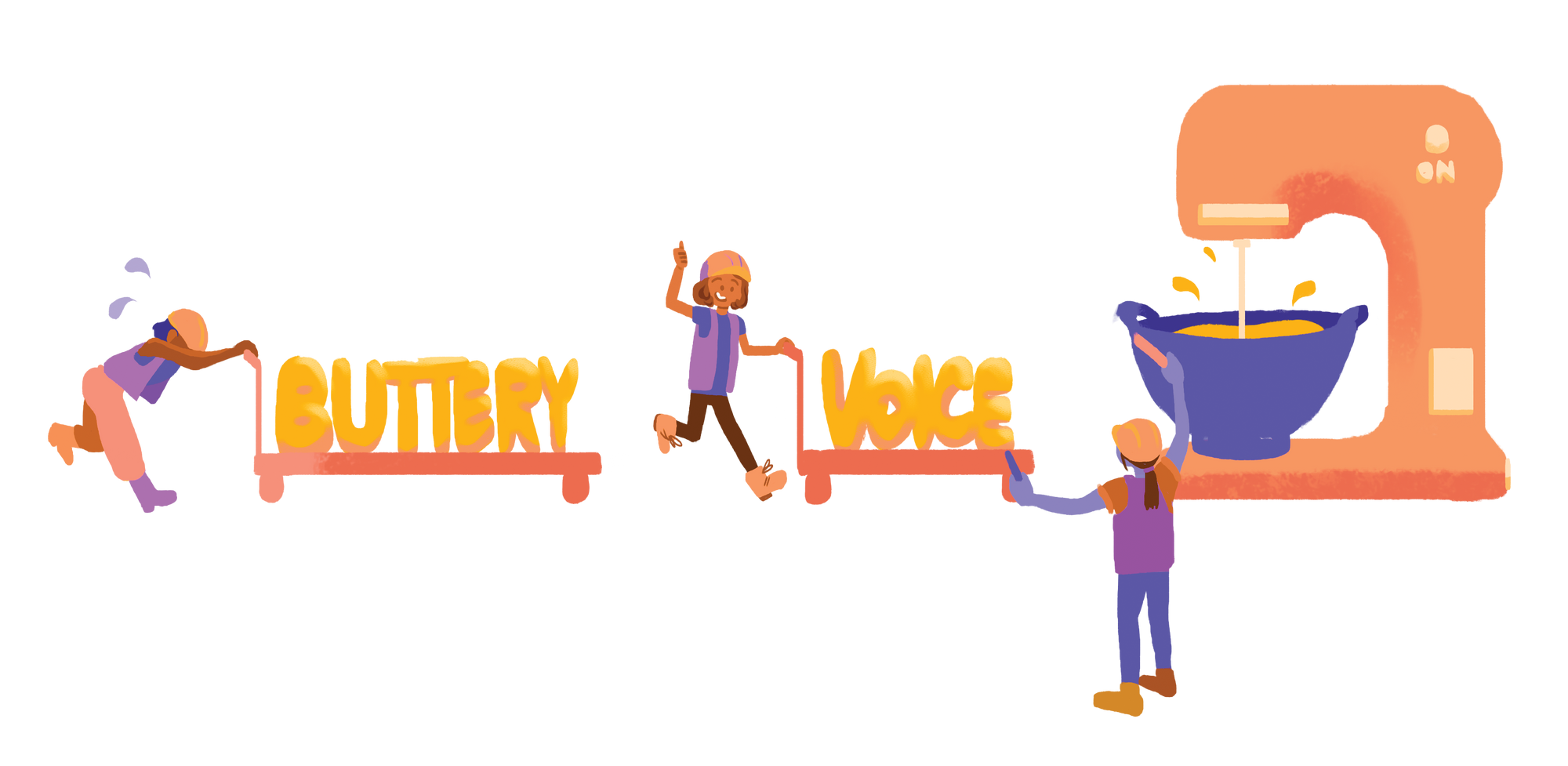
The Cortex as a Dynamic Unit:
In order to understand language processing, it is important to have a firm grasp on the function of our frontal cortex. The cortex is the outer layer of the cerebrum primarily composed of grey matter (neuron cell bodies) and is divided into two parts by the longitudinal fissure that separates the left and right hemispheres. It is responsible for memory, perception, attention, thoughts, language, and consciousness and is considered to play a crucial role in the way our sensory and motor neurons create associations between words. Donald Hebb, a Canadian psychologist who pioneered the idea of neural webs, defines the cortex as a network of neurons processing stimuli from various input and output sources [1]. Recent research has revealed the cortex is not a static area of the brain that operates independently, but rather an “information mixing device” in which one neuron can carry information from many different sources [1]. This is in direct association with what Hebb calls “correlation learning,” a phenomenon where two or more systems of cells that are active at the same moment consistently become “associated” with one another so that activity stimulating one cell will stimulate the other [1]. Some words associated with those senses cause these cells to detect stimuli in those areas, which is known as multisensory integration. For example, when reading “buttery” and “voice,” cells associated with food will be stimulated at the same time as those for recognizing sounds and the word “voice.” If this pattern is continued over time, the two words will automatically be associated with one another in the brain – known as neural efficiency [1]. As Hebb stated, “neurons that fire together wire together” [2]. In this way, our cortex acts as an information-mixing device that creates connections between different neurons and between different words and stimuli.
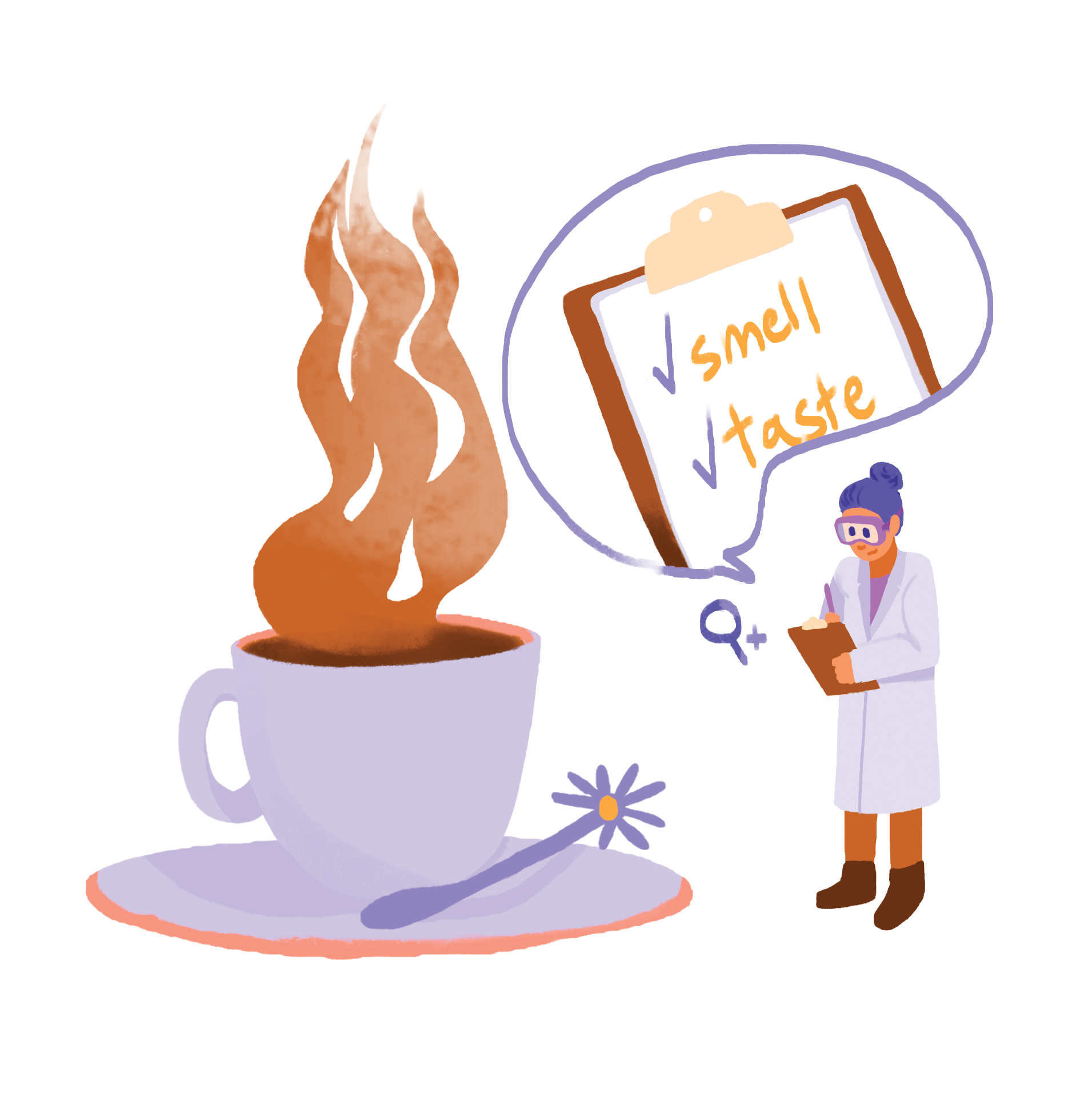
Word Webs and Functional Webs:
According to Hebb’s view, the cortex is composed of multiple functional webs, or networks of neurons from various sources, that carry associated information and reassembles it to create new information. For example the smell of coffee, the taste of coffee, and the texture of the foam on top of the coffee is combined to create our perception of coffee [1]. This idea implies that if, for example, one part of the web is impaired, the corresponding part of the neuronal web will also be dysfunctional. An experiment to test this hypothesis was conducted on Macaque monkeys, where either the prefrontal cortex or the inferior temporal lobe was cooled while the monkeys were presented with different colored shapes. While this study focused on colors and shapes, this still demonstrates the functioning of word-webs. Cooling one part of the brain by applying very cold temperatures over a short period of time to decrease neuronal firing activity, known as Targeted Temperature Management (TTM), caused the other part of the brain to also lose complete activity, implying that the two were directly associated with each other through a neuronal web. The monkeys who did not have their neuronal networks cooled displayed neuronal firing activity in the prefrontal cortex and in the inferior temporal lobe simultaneously, only firing when the exact combination of associated color and shape were shown [1]. A similar experiment, also done on Macaque monkeys, demonstrated that a sector of mirror neurons F5 in monkeys is analogous to the area in humans in the inferior frontal cortex, which is responsible for action imagery and language understanding [3-5]. Mirror neurons are neurons that fire both when an animal acts and observes the same action performed by another individual [3-5]. Similarly, patients that had diseases affecting the motor nervous system, such as motor neuron disease, had greater difficulty in processing action-related verbs [6]. These studies support the hypothesis that more than one area of the brain processes and associates words, and that impairing or stimulating one part of the brain affects the other. Word webs would primarily consist of connections between neurons storing word formation data and neurons involved in processing activities and perceptions.
A more recent experiment also supported the theory of word webs and associated learning. Dr. Yury Shtyrov and his research team from the University of Cambridge hypothesized that words involving movements of different body parts should incorporate neurons in different parts of the sensorimotor cortices [2]. In order to test their hypothesis, they addressed brain activity related to semantic processing using a technique called mismatch negativity or MMN. This method allowed the researchers to investigate long-term memory traces for language elements in the brain. The MMN has recently been shown to demonstrate and reflect memory traces for language elements such as phonemes (units of sound that distinguish one word from another, like t and r in cat and car), syllables, and morphemes (collection of phonemes). In this particular experiment, the researchers observed the processing of words referring to movements with different body parts – these words were pick and kick. The researchers specifically chose these words because sometimes it is extremely difficult to distinguish between activity due to physical stimuli activating a region of the brain or semantic stimuli; therefore it is important for both words to have the same phoneme endings. The group subjected thirteen individuals to a behavior evaluation where fragments of each word were presented to the individual one at a time and each person was asked to name each word or syllable that was identifiable. Through this experiment, the researchers noticed a fascinating pattern: pick was found to activate centroposterior parts of the brain and kick was found to activate lateral parts of the brain, which was contrary to their predicted results [2]. This is significant because it supports the theory that different locations of the brain are semantically related to body movements; the fact that pick activated a larger region is logical considering it can involve more than one movement and more than one part of the body, unlike kick. These conclusions are consistent with the view that words are encoded in the brain by distributed neural networks [2]. According to various experimental data performed on this topic, associations related to different words, such as action and content words, are processed in different locations of the brain, but the connections between associations and words span the entire brain in a complex neuronal network [2].
Research on how different words are perceived and processed in various parts of the brain categorized words into two groups: content and function words [7]. Content words are typically nouns, adjectives, or verbs. They are frequently associated with a physical object, such as table or cat. Upon encountering such a word in our daily lives, an episodic memory event may occur, where a particular memory from our past is associated with that word (such as connecting the word school with the physical activity of recess from first grade memories). Functional words, however, have linking purposes in words such as although or because. Through fMRI experiments that measured these episodic memory events in individuals, researchers noticed distinct lateral word processing between content and functional words. Sometimes, multiple parts of the brain would simultaneously light up when exposed to a content word or functional word, providing further evidence that the cortex is much more akin to a web rather than a rigid information processor [7].
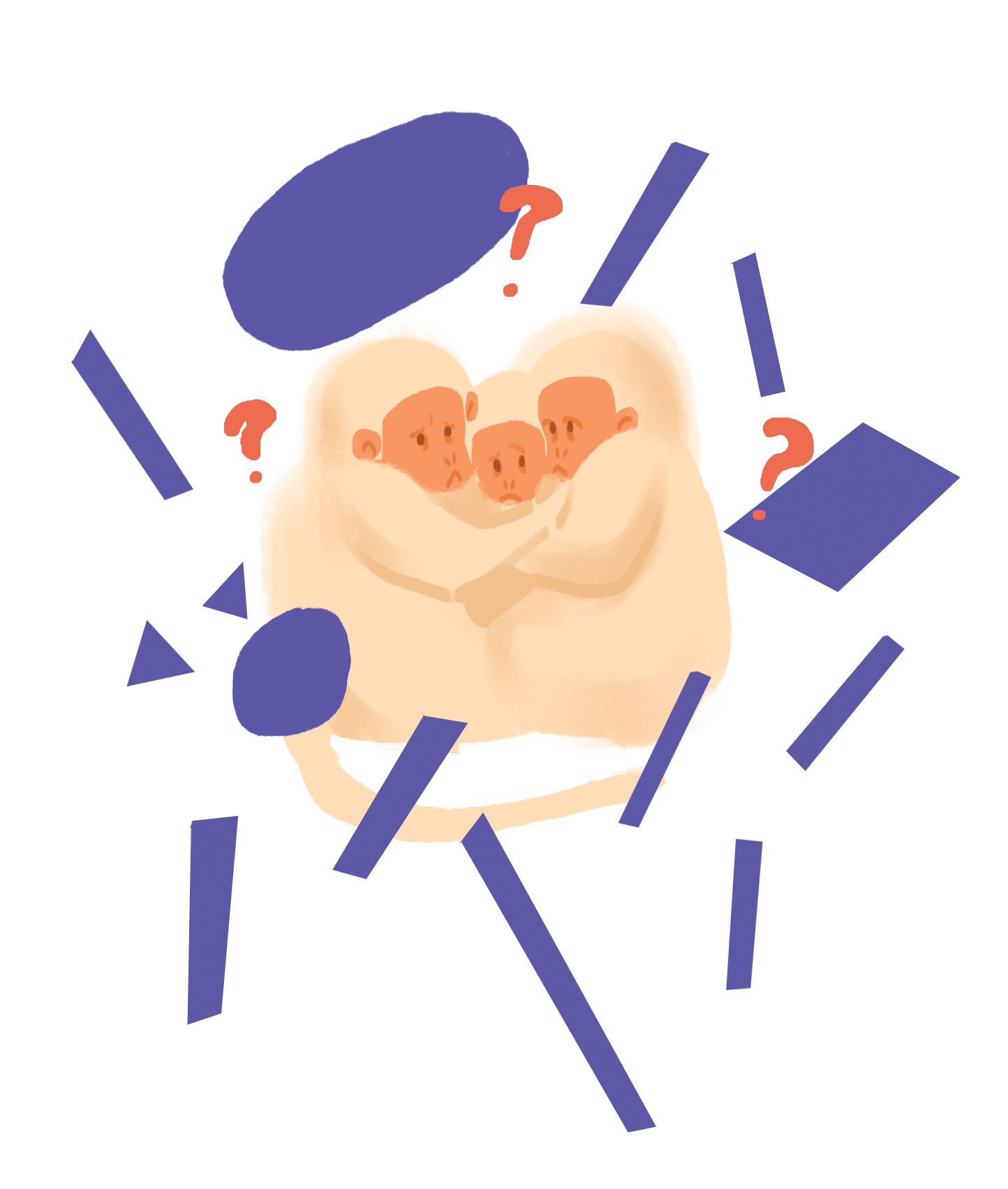
Words and Emotions:
So how are we capable of taking all these words and experiencing different emotions and imagining different scenarios? There are two sensations that distinguish our perception of words: valence and arousal. Valence is the amount of pleasure one gets from a word, whereas arousal is a measure of how willingly one would want to approach or escape from the word, similar to the “fight-or-flight response” we might experience when we encounter a word [8-18]. There are two prevailing theories on how our brains are capable of emotionally processing words. Arousal theory states that the right hemisphere is more adept at processing words than the left, primarily supported by studies conducted on individuals with one hemisphere of their brain impaired, as presented with the macaque monkey study. Valence theory states that both hemispheres are active during emotional processing but to different extents depending on the valence of a certain word. There is, however, a third emerging theory called the circumplex theory, which combines the two aforementioned theories into one unified theory that analyzes both the amount of valence a word creates and the amount of arousal. Recent research by Dr. Moscovitch, a professor of psychology at the University of Toronto, claims that memory of a word is processed in the right portion of the brain whereas perception of a word is composed in the left, and together they create a complete picture of a word [8-18]. Continued studies show further evidence that emotional processing of words is not restricted to one or the other hemisphere; rather, both process it, which is more akin to a web-like model.
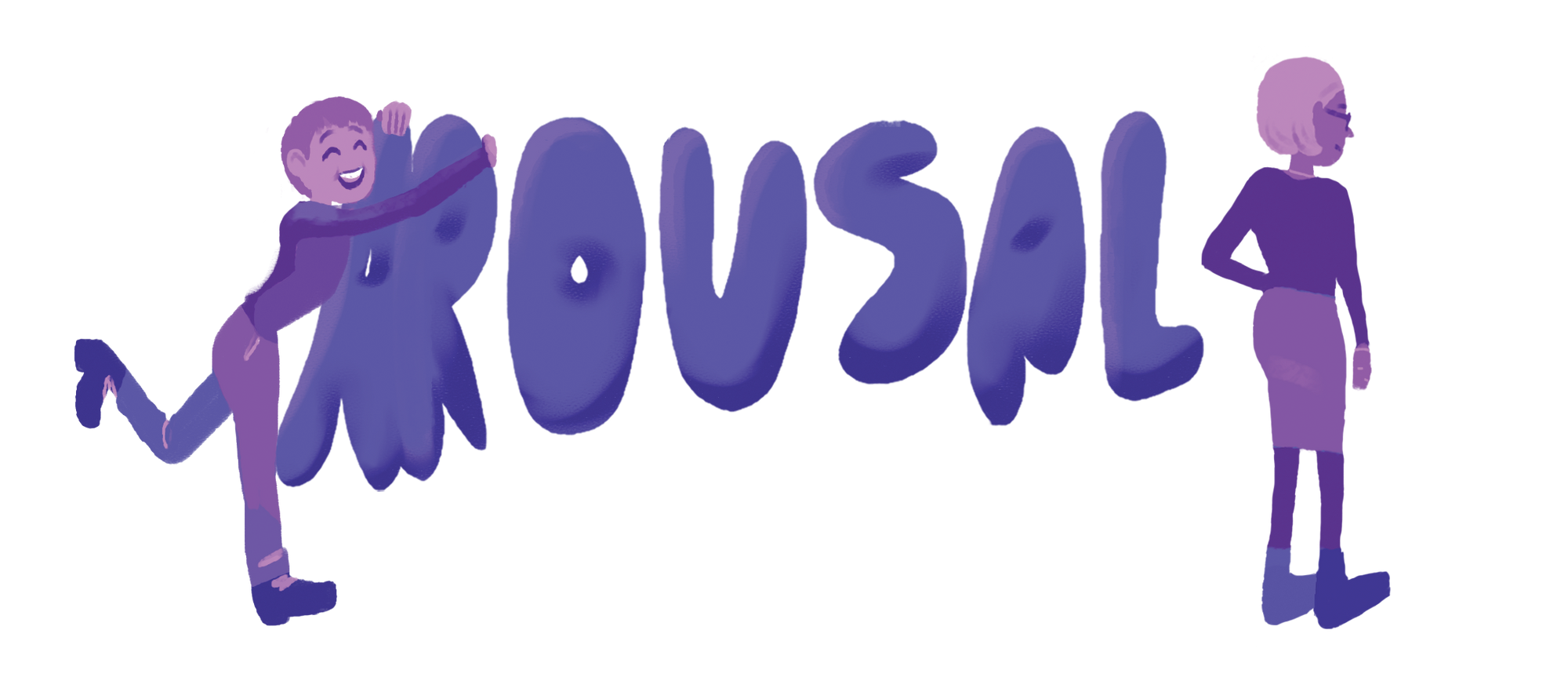
New Views:
Other recent research has introduced a new concept of word processing that incorporates the previous idea of word webs, but presents the sensory system as an input system that filters emotion and memory associated with a word and in effect dictates the actions of the motor system [19, 20]. In other words, the posterior portion of the cortex serves as a sensory information filter, and the frontal motor primarily functions under the control of the cognitive, posterior cortex system. This idea emerged after a neuroimaging experiment that studied specific motor activations by directing subjects to distinguish between speech sounds, word meanings, and sentence structures. Individuals with lesions in the inferior frontal regions of the brain, which are responsible for motor circuits involved in the comprehension of grammar and sentence structure, demonstrated greater difficulty than those who did not have lesions [19, 20]. This observation also supports previous hypotheses about word webs and neuronal associations between different parts of the brain [1].
Conclusion:
Everyday language processing proves to be much more complicated than previously conceived. While we take in the multitude of verbal messages we encounter every day in the form of fliers, conversations, lectures, and books, our brain is busy making neural connections between different parts of the brain so if we ever encounter the word again, we have a specific memory or association coupled with it. For instance, the word “geology” might immediately recall the memory of learning geology in a classroom for one person or actually doing specific research on geology for another. It appears different people may perceive words differently from each other due to the neuronal word webs they develop that associate their personal experiences with specific words.
References:
- Diaz, M. T., & Mccarthy, G. (2009). A comparison of brain activity evoked by single content and function words: An fMRI investigation of implicit word processing. Brain Research, 1282, 38-49.
- Shtyrov, Y., Hauk, O., & Pulvermüller, F. (2004). Distributed neuronal networks for encoding category-specific semantic information: the mismatch negativity to action words. European Journal of Neuroscience, 19(4), 1083-1092.
- Hubel, D. Eye, Brain, and Vision (Scientific American Library, New York, 1995).
- Fadiga, L., Fogassi, L., Pavesi, G. & Rizzolatti, G. Motor facilitation during action observation: a magnetic stimulation study. J. Neurophysiol. 73, 2608–2611 (1995).
- Fadiga, L., Craighero, L., Buccino, G. & Rizzolatti, G. Speech listening specifically modulates the excitability of tongue muscles: a TMS study. Eur. J. Neurosci. 15, 399–402 (2002).
- Wittgenstein, L. Philosophical Investigations (Blackwell Publishers, Oxford, 1953).
- Pulvermüller, F. (2001). Brain reflections of words and their meaning. Trends in Cognitive Sciences, 5(12), 517-524.
- Caramazza, A. (1996). Pictures, words and the brain. Nature, 383(6597), 216-217.
- Caramazza, A. and Zurif, E.B. Lang. 3, 572-582 (1976).
- Vandenberghe, r. Price, C., Wise, R., Josephs, O. And Frackowiak, R. S. J. Nature 383, 254-256 (1996).
- Damasio, H., Grabowski, T.J., Tranel, D., Hichwa, R.D. and Damasio, A.R. Nature 380, 499-505 (1996).
- Beauvois, M.F. Phil. Trans. R. Soc. Lond. B 298, 35-47 (1982).
- Shallice, T. in The Cognitive Neuropsychology of Language (eds Coltheart, M., Sartori, G. and Job, R.) 111-127 (Lawrence Erlbaum, Hove and London, 1987).
- Coslett, H.B. and Saffran, E.M. Brain 112, 1091-110 (1989).
- Caramazza, A., Hillis, A.E., Rapp, B. and Romani, C. Cogn Nueropsychol. 7, 161-189 (1990)
- Riddoch, M. J., Humphreys, G.W., Coltheart, M. and Funnell, E. Cogn. Neuropsychol. 3, 3-25 (1988).
- Warrington, E. K. and Shallice, T. Brain 107, 829-852 (1984).
- Martin, A., Wiggs, C., Ungerleider, L. and Haxby, J. Nature 379, 649-652 (1996).
- Pulvermüller, F., Shtyrov, Y. & Ilmoniemi, R. J. Spatio-temporal patterns of neural language processing: an MEG study using minimum-norm current estimates. Neuroimage 20, 1020–1025 (2003).
- Pulvermüller, F. et al. Motor cortex maps articulatory features of speech sounds. Proc. Natl Acad. Sci. USA 103, 7865–7870 (2006).