In recent years, the scientific community has increasingly been going with its gut. That is to say, scientists have been looking to the human gastrointestinal microbiota for its role in metabolism, health, and disease. The existence of a close functional relationship between gastrointestinal microbiota and the gastrointestinal tract is somewhat intuitive, but less intuitive is the intriguing connection between microbiota and the central nervous system. Recent studies have demonstrated that the profound influence of the human microbiota on the functioning and health of the body extends also to the brain, with significant implications for neurological diseases and nervous system development.
WHAT IS THE HUMAN MICROBIOTA?
Modern research of host-associated microbial communities has been made possible by advances in microbial research techniques that were made in the 1980s [1,2]. Previously, research of bacteria had largely been accomplished through analysis of pure cultures of bacteria grown in a Petri dish; however, bacteria in culture are generally not representative of the rich diversity of bacterial species present in a given environment, such as the gastrointestinal microbiota. On top of this, many bacterial species – including most of the microbes that populate the human gastrointestinal tract – are difficult to culture [3]. These shortcomings of culture techniques eventually gave rise to the development of techniques for studying uncultured bacteria, including ribosomal RNA sequencing techniques as well as techniques for directly cloning and analyzing DNA from environmental samples, called metagenomics [1, 2]. Armed with these methods, the scientific community has gained vast insight into the nature and impacts of host-associated microbes through studies such as the Human Microbiome Project, which was established in 2007 to sequence and analyze the health impacts of the human microbiota [4].
The characteristics of the human microbiota are remarkable and perplexing. It is an incredibly diverse biome, consisting not only of a variety of bacterial species but also of the occasional virus and protozoan [5]. The human microbiota generally consists of approximately 100,000,000,000,000 (that’s 100 trillion) bacterial cells, thus accounting for 90% of the cells in the human body [1]. The largest, most widely studied community of microbes can be found living in the lower gastrointestinal tract [5, 6]; however, microbes also populate our mouth, our inner elbows, and even the creases behind our ears [7]. The species of microbes which are present at each site vary between different individuals. While all humans share a core microbiome, variation in microbiome composition depends on factors such as diet, genetics, and even recent kissing partners [8]. Based on genomic analysis from the Human Microbiome Project, some fundamental metabolic functions are common to all microbes populating humans whereas some metabolic functions are exclusive to single microbial species and are uniquely useful to the human host [7]. The functions of genes in the microbiome require further study, as the vast majority (78-86%) of genes present in our body’s large microbial communities have unknown functions [7].
For the most part, the relationship between humans and their microbial tenants could be described as mutualistic, meaning that both organisms benefit from the interaction. Bacteria residing in the intestine help their human hosts by synthesizing and excreting vitamins, completing metabolic functions that human cells cannot perform, preventing pathogen colonization in the intestine, producing chemicals necessary to stimulate epithelial cell growth and metabolism, and stimulating the development of the immune system. The role of bacteria in maintaining human health is further demonstrated by the fact that imbalances in microbiota composition, called dysbiosis, have been found to be associated with numerous diseases [5]. Some of the diseases with the most well-characterized connections to dysbiosis include obesity and gastrointestinal diseases such as Crohn’s disease, ulcerative colitis, irritable bowel syndrome, and colorectal cancer [5]. In the latter half of this last decade, research in the subject area of the microbiota and human health has also begun to explore the relationship between the microbiota and the central nervous system, yielding exciting insights into both neurological diseases and brain development [6].
THE LINK BETWEEN THE GASTROINTESTINAL MICROBIOTA AND THE CENTRAL NERVOUS SYSTEM
To discuss the relationship between the gastrointestinal microbiota and the central nervous system, it is critical to first understand the nature of the gut-brain axis. The gut-brain axis is a bidirectional system of communication which connects the gastrointestinal tract to the central nervous system via endocrine, immunological, and neuronal signaling [6]. The gastrointestinal tract is innervated by both the sympathetic nervous system, which activates the fight-or-flight-response, and the parasympathetic nervous system, which regulates involuntary bodily functions [9]. Through these connections as well as hormonal signaling, the brain regulates functions such as secretion, motility, intestinal permeability, and immune responses in the gastrointestinal tract [6, 9]. In turn, the brain receives information from the gastrointestinal tract via afferent neural pathways (i.e., neural pathways where signals travel from the body to the brain) and via chemical signaling in the form of hormones and signaling proteins called cytokines which are released from immune cells [6].
Microbes in the gastrointestinal tract putatively affect this latter leg of the gut-brain axis when they release molecules as messages or metabolic products [9]. These molecules may influence the central nervous system by acting on gastrointestinal immune and endocrine cells, entering the bloodstream and then the brain (through structures representing “chinks” in the blood-brain barrier, such as the area postrema), or influencing signaling in neural pathways going to and from the central nervous system [6, 9]. One especially influential study, produced collaboratively by researchers at University College Cork in Ireland and McMaster University in Canada, provided strong evidence that the microbiota influences the brain via afferent neuronal pathways. In this study, it was found that an excess of the bacterium Lactobacillus rhamnosus in the gastrointestinal tract caused modified behavior and altered gene expression in the brain only when the vagus nerve, the key afferent nerve connecting the gastrointestinal tract to the brain, was present [10]. Additionally, other studies have supported the ability of chemicals produced by gastrointestinal microbiota to travel to and directly influence the central nervous system [11, 12].
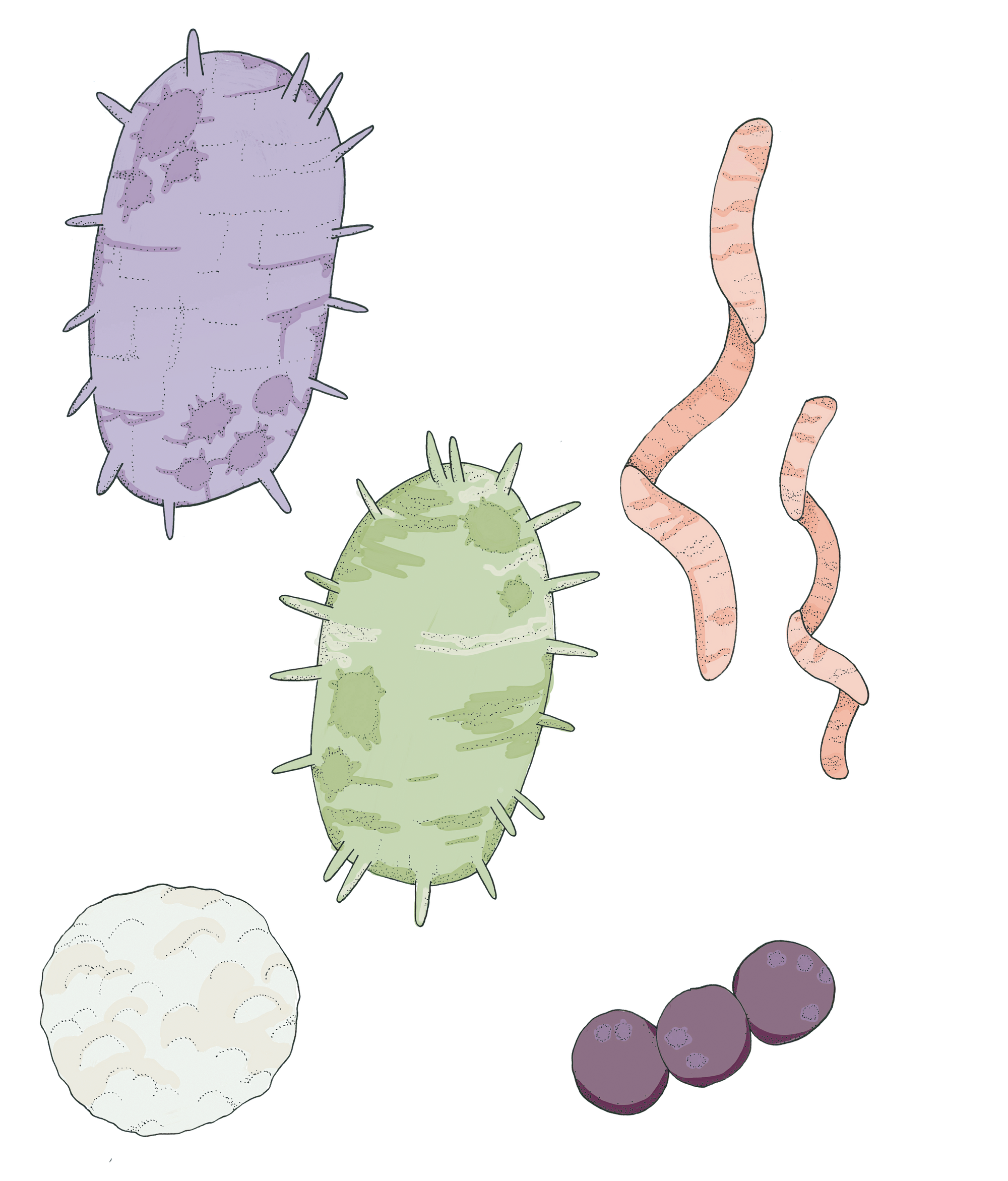
IMPLICATIONS FOR BRAIN DEVELOPMENT AND CENTRAL NERVOUS SYSTEM DISEASES
This influence of the microbiota on the gut-brain axis appears to be the mechanism whereby dysbiosis contributes to the progression of central nervous system diseases. A few of the central nervous system diseases that have most prominently exhibited associations with gastrointestinal dysbiosis are autism spectrum disorder, Parkinson’s disease, and multiple sclerosis [6]. Numerous studies have demonstrated characteristic abnormalities in the microbiota composition of the gastrointestinal tract of children with autism, which align with previously noted abnormalities in blood metabolite concentration [12, 13]. A 2016 study by scientists at the California Institute of Technology demonstrated in a mouse model that Parkinson’s disease could only occur when gastrointestinal microbes are present and that symptoms of the disease could be worsened in mice upon colonization with gastrointestinal microbiota of a human Parkinson’s disease patient [14]. Abnormal trends in gastrointestinal microbiota composition have also been observed in multiple sclerosis [11]. However, in many cases of demonstrated connection and even contribution of the microbiota to neurological diseases, the reason for the association is poorly understood [5].
The effect of the microbiota on the brain has more permanent consequences in the context of development. The conditions of the brain – and of all organs – during development are critical because their effect on development will be reflected in the functioning of the organ throughout an individual’s entire life, a phenomenon called developmental programming [15]. In one study, researchers in Japan examined of the effect of microbiota on the development of the hypothalamic-pituitary-adrenal (HPA) axis, a neuroendocrine system which controls the physiological response to stress [16]. The researchers first identified that mice lacking microbiota, called germ-free mice, exhibited an exaggerated HPA response to stress relative to mice with normal functional microbiota. They then colonized the germ-free mice with normal microbiota to determine whether the HPA response to stress in the germ-free mice could be normalized. They found that colonization with normal microbiota at a young age resulted in partial normalization of the HPA stress response in germ-free mice whereas colonization at a later stage of development did not result in normalization of the HPA stress response. A similar result was found in a study conducted by researchers in Sweden in 2011, wherein it was found that certain differences in anxiety-related behaviors between germ-free mice and mice with a normal microbiota could be normalized by colonizing germ-free mice with normal gastrointestinal microbiota at a young age but not at a later developmental stage [15]. These studies are significant because they demonstrate that the state of the gastrointestinal microbiota during development can cause permanent changes in the physiological functioning and gene expression of the brain.
The implications that this demonstrated relationship between microbiota on brain development could have for humans are quite profound. Studies have shown that the development of the gastrointestinal microbiota in humans is an entirely postnatal process that begins with maternal microbiotic transmission via breastfeeding and lasts for 2-3 years [17, 18]. Malnutrition during those early years of life causes the development of the gastrointestinal microbiota to be delayed. As such, the gastrointestinal microbiota of undernourished children does not fully reach a mature configuration and is not sustainably brought to a mature configuration by therapeutic food interventions, which are common treatments for malnutrition [19]. Given the relationship between brain development and the composition of the microbiota, some scientists hypothesize that this persistent immaturity of the gastrointestinal microbiota could contribute to the impaired cognition observed during adulthood in severely malnourished individuals. However, extensive research into this subject has yet to be conducted [18].
CONCLUSION
Inquiry into the connection between neurological health and the gastrointestinal microbiota is an exciting endeavor because it affords intriguing opportunities to develop novel therapeutic interventions. For example, scientists have just begun to explore in greater depth the application of probiotics – microbes which are administered for a health benefit – to psychiatric disorders. One systematic review published in the Journal of Neurogastroenterology and Motility in October 2016 assessed 38 published studies of the positive effects of probiotics on central nervous system disorders, demonstrating that probiotics have been successfully used to ameliorate symptoms of depression, anxiety, autism spectrum disorder, and obsessive-compulsive disorder [20]. Additionally, some scientists encourage the application of fecal microbiota transplantation – a treatment in which microbiota are transplanted from a healthy donor to a patient in the form of feces – to neuropsychiatric disorders associated with dysbiosis. The technique has been successfully used in treating a number of gastrointestinal disorders, and several studies have been conducted in the past few years on the use of fecal microbiota transplants to treat Parkinson’s disease, multiple sclerosis, and autism [21, 22].
Given the burgeoning research currently surrounding the relationship between the gastrointestinal microbiota and the brain, these innovative treatment approaches certainly represent an appealing and promising approach to addressing neurological conditions. However, continued progress in this field will require overcoming a number of limitations on our current understanding of the gastrointestinal microbiota. For instance, scientists do not currently have clear parameters for defining what a healthy or normal gastrointestinal microbiota configuration looks like [3]. Additionally, due to the complexity of intermicrobial and host-microbe interactions within host-associated microbiota, it is difficult to pinpoint the effects of imbalances in the abundances of individual species on the host. Taken together, these limitations have made it difficult to progress from researching correlations between diseases and microbiotic imbalances to researching the possible causal role of the microbiota in certain diseases. In order to move past these barriers, further methodological and technological advancement and innovative applications of current research techniques will be required [3]. However, with such technological advances being within reach, further research in this exciting and fast-paced field of study promises to yield greater insight into the role of the microbiota in neurological diseases, development, and health.
References
1. Robinson, C. J., Bohannan, B. J., & Young, V. B. (2010). From Structure to Function: the Ecology of Host-Associated Microbial Communities. Microbiology and Molecular Biology Reviews, 74(3), 453-476. doi:10.1128/mmbr.00014-10
2. Riesenfeld, C. S., Schloss, P. D., & Handelsman, J. (2004). Metagenomics: Genomic Analysis of Microbial Communities. Annual Review of Genetics, 38(1), 525-552. doi:10.1146/annurev.genet.38.072902.091216
3. Van den Elsen, L. W., Poyntz, H. C., Weyrich, L. S., Young, W., & Forbes-Blom, E. E. (2017). Embracing the gut microbiota: the new frontier for inflammatory and infectious diseases. Clinical & Translational Immunology, 6(1), e125–. http://doi.org/10.1038/cti.2016.91
4. Human Microbiome Project: Overview. (n.d.). Retrieved January 16, 2017, from https://commonfund.nih.gov/hmp/overview
5. Biedermann, L., & Rogler, G. (2015). The intestinal microbiota: its role in health and disease. European Journal of Pediatrics, 174(2), 151-167. doi:10.1007/s00431-014-2476-2
6. Collins, S. M., Surette, M., & Bercik, P. (2012). The interplay between the intestinal microbiota and the brain. Nature Reviews Microbiology, 10(11), 735-742. doi:10.1038/nrmicro2876
7. Human Microbiome Project Consortium. (2012). Structure, function and diversity of the healthy human microbiome. Nature 486, 207–214
8. Kort, R., Caspers, M., Graaf, A. V., Egmond, W. V., Keijser, B., & Roeselers, G. (2014). Shaping the oral microbiota through intimate kissing. Microbiome, 2(1), 41. doi:10.1186/2049-2618-2-41
9. Mayer, Emeran A., Tillisch, Kirsten, Gupta, Arpana. (2015) Gut/brain axis and the microbiota. J Clin Invest. 2015, 125(3), 926-938. doi:10.1172/JCI76304.
10. Bravo, J. A., Forsythe, P., Chew, M. V., Escaravage, E., Savignac, H. M., Dinan, T. G., . . . Cryan, J. F. (2011). Ingestion of Lactobacillus strain regulates emotional behavior and central GABA receptor expression in a mouse via the vagus nerve. Proceedings of the National Academy of Sciences, 108(38), 16050-16055. doi:10.1073/pnas.1102999108
11. Bhargava, P., & Mowry, E. M. (2014). Gut Microbiome and Multiple Sclerosis. Current Neurology and Neuroscience Reports, 14(10). doi:10.1007/s11910-014-0492-2
12. Bolte, E. (1998). Autism and clostridium tetani. Medical Hypotheses, 51(2), 133-144. doi:10.1016/s0306-9877(98)90107-4
13. Finegold, S. M., Downes, J., & Summanen, P. H. (2012). Microbiology of regressive autism. Anaerobe, 18(2), 260-262. doi:10.1016/j.anaerobe.2011.12.018
14. Sampson, T., Debelius, J., Thron, T., Janssen, S., Shastri, G., Ilhan, Z., . . . Mazmanian, S. (2016). Gut Microbiota Regulate Motor Deficits and Neuroinflammation in a Model of Parkinson’s Disease. Cell, 167(6). doi:10.1016/j.cell.2016.11.018
15. Heijtz, R. D., Wang, S., Anuar, F., Qian, Y., Bjorkholm, B., Samuelsson, A., . . . Pettersson, S. (2011). Normal gut microbiota modulates brain development and behavior. Proceedings of the National Academy of Sciences, 108(7), 3047-3052. doi:10.1073/pnas.1010529108
16. Sudo, N., Chida, Y., Aiba, Y., Sonoda, J., Oyama, N., Yu, X.-N., … Koga, Y. (2004). Postnatal microbial colonization programs the hypothalamic–pituitary–adrenal system for stress response in mice. The Journal of Physiology, 558(Pt 1), 263–275. http://doi.org/10.1113/jphysiol.2004.063388
17. Fouhy F, Ross RP, Fitzgerald GF, Stanton C, Cotter PD. Composition of the early intestinal microbiota: Knowledge, knowledge gaps and the use of high-throughput sequencing to address these gaps. Gut Microbes. 2012;3(3):203-220. doi:10.4161/gmic.20169.
18. Goyal M. S., Venkatesh S., Milbrandt J., Gordon J. I., Raichle M. E. (2015). Feeding the brain and nurturing the mind: linking nutrition and the gut microbiota to brain development. Proc. Natl. Acad. Sci. U.S.A., 112, 14105–14112. 10.1073/pnas.1511465112
19. Subramanian, S., Huq, S., Yatsunenko, T., Haque, R., Mahfuz, M., Alam, M. A., … Gordon, J. I. (2014). Persistent Gut Microbiota Immaturity in Malnourished Bangladeshi Children. Nature, 510(7505), 417–421. http://doi.org/10.1038/nature13421
20. Wang, H., Lee, I., Braun, C., & Enck, P. (2016). Effect of Probiotics on Central Nervous System Functions in Animals and Humans: A Systematic Review. Journal of Neurogastroenterology and Motility, 22(4), 589-605. doi:10.5056/jnm16018
21. Choi, H. H., & Cho, Y. (2016). Fecal Microbiota Transplantation: Current Applications, Effectiveness, and Future Perspectives. Clinical Endoscopy, 49(3), 257-265. doi:10.5946/ce.2015.117
22. Xu, M. (2015). Fecal microbiota transplantation broadening its application beyond intestinal disorders. World Journal of Gastroenterology, 21(1), 102. doi:10.3748/wjg.v21.i1.102