Recent advances in neuroscience have revealed the stunning level of diversity in the human brain: virtually every neuron is genetically unique due to hundreds of mutations present in each cell [1][2][3]. Let that sink in – every thinking, functioning human brain contains hundreds of random genetic errors per neuron. These errors are the result of somatic mutations, which are changes that are not inherited from a parent and are only present in a subset of cells. Tissues containing somatic mutations are said to possess mosaicism. Picture the tissue as a tiling of cells with different genetic profiles, analogous to the multicolored tiles of a mosaic art piece. While the vast majority of somatic mutations are inconsequential, a small percentage of them impact how our brains develop and function. These mutations occur continuously and accumulate with age, so an octogenarian has thousands of such mutations in every neuron [2]. We now know that the mosaic brain is even more genetically diverse than previously thought, and this may have fascinating implications for human individuality.
The useful technique of single cell sequencing has been instrumental in recent discoveries about neuronal genetic diversity. This technique is exactly what it sounds like: it determines the genetic sequence of individual cells. Single cell sequencing is especially useful for observing somatic mutations, as each one may only be present in an individual cell or a small group of cells.
A study conducted by Taejeong Bae and colleagues at the Mayo Clinic used single cell sequencing to investigate mosaicism in the developing brain. The researchers sequenced 31 single cells from a neuron-producing region of 15- to 21-week-old fetal, post-mortem brains [1]. They were able to recognize somatic mutations unique to the nervous system by comparing the genetic sequences of neurons to those of cells from the spleen. The study found that on average, there were already 200 to 400 mutations per cell at this fairly early stage of development. This corresponds to a mutation rate that is about 50 times higher than that of stem cells in the liver and small intestine. Thus, by the time a human is born, the brain contains an incredible amount of genetic diversity among its 100 billion cells [1].
Neural genetic diversification does not stop at birth. A second study investigated mutations that occur from infancy into late adulthood [2]. A team of researchers led by Michael Lodato at Harvard University used single cell sequencing to analyze the brains of individuals aged four months to 82 years. They discovered that the number of somatic mutations per cell positively correlates with age, and that by the time a neuron is 80 years old, it has accumulated around 2,000 to 4,000 somatic mutations. This leads to the astonishing conclusion that every neuron is genetically unique—our brains are thoroughly heterogeneous mosaics [2].
Mosaicism can result in important differences among cells, but not all mutations have a functional consequence. Out of the billions of bases contained in the human genome, only relatively small stretches contain “instructions” for the construction and regulation of proteins. For this reason, mutations rarely impact gene products. The Harvard team estimated that about one in 1,000 neurons will gain a somatic mutation that precludes the normal function of a gene and thereby alters the cell in some way [2]. This means that the mind is even more complex than previously thought. Given the random nature of somatic mutations, there are innumerable possibilities for the genetic profile of an individual brain.
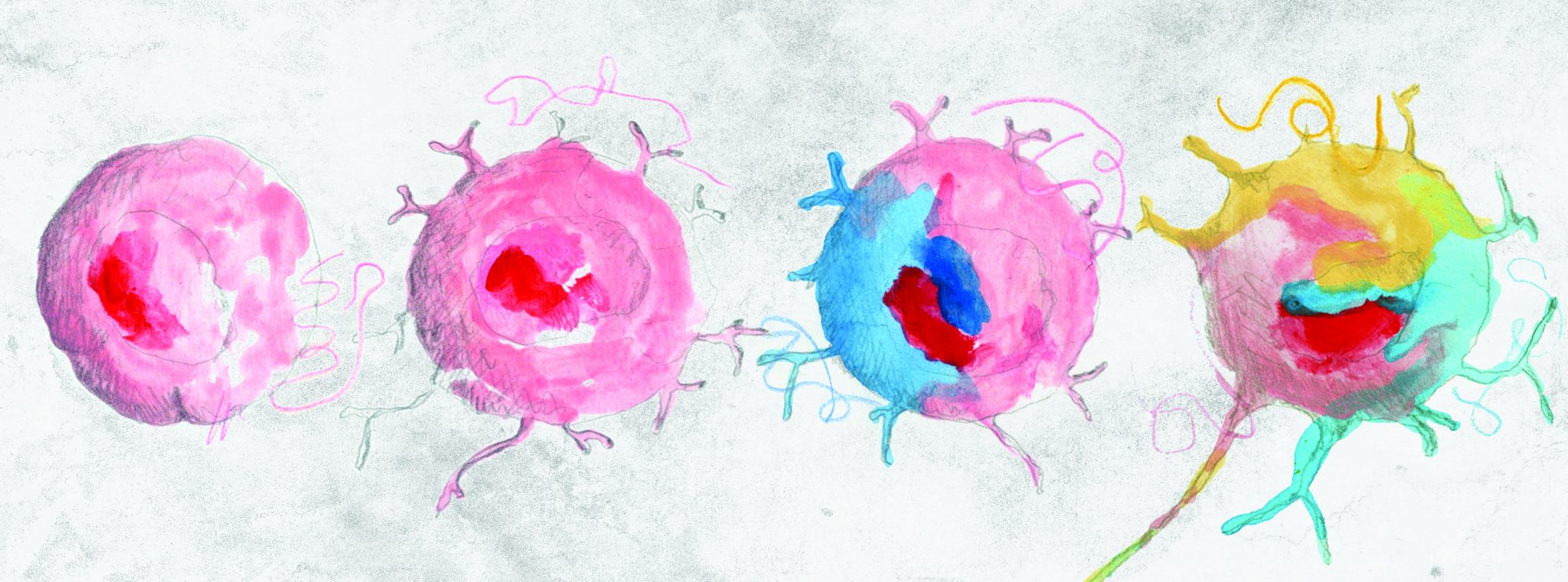
The idea that each human brain is distinct and ever-changing may provide us with a new means of explaining human individuality. Decades ago, research established that genetics strongly influence personality: identical twins, who have the exact same genome but some differences in their early life environments, share many of the same traits. Any trait disparities between twins have traditionally been explained by those environmental differences or non-shared experiences [4][5]. More recently, scientists have discovered a third personality-modulating element in epigenetics, which are factors that turn genes “on” or “off" [6]. Epigenetic marks can have functional consequences similar to mutations, though they involve no changes in the sequence of the genetic code. Now, given the findings from Harvard and the Mayo Clinic, it seems possible that somatic mutations are an additional component that could influence personality.
Somatic mutations have already been shown to be associated with the development of aberrant, disease-related behaviors. For example, somatic mutations in a particular gene are often found in children with epilepsy [7]. One study found that introducing mutations to this gene in a small number of cells in a mouse brain was sufficient to cause intractable epilepsy [8]. This suggests that somatic mutations, each of which likely affects only a minute fraction of brain cells, can disrupt normal function at a brain-wide level. Other studies have suggested somatic mutations play a role in autism, schizophrenia, and major depressive disorder [9]. Researchers speculate that somatic mutations may cause these phenotypes by disrupting neural connectivity.
If somatic mutations in the brain can cause disease-related behaviors, it is possible that they could more subtly influence behavior by affecting the neural circuitry that underlies personality traits. Consider this hypothetical example: connections between the prefrontal cortex and the amygdala (commonly referred to as the “emotional center” of the brain) are thought to be involved in normal responses to emotionally-charged stimuli [10]. Certain somatic mutations occurring in stem cells during brain development could alter the wiring of this circuit or decrease the function of many key neurons in this pathway since these cells pass their genes to many daughter neurons. In this case, the individual could be more likely to display emotionlessness or callousness. Though there is currently no published scientific evidence supporting this particular mechanism, it seems entirely possible that somatic mutations could influence traits in this way. As there are known brain circuits and regions related to mood, attention, language, and many other functions, it is conceivable that somatic mutations could modulate various traits or behaviors to a spectrum of degrees.
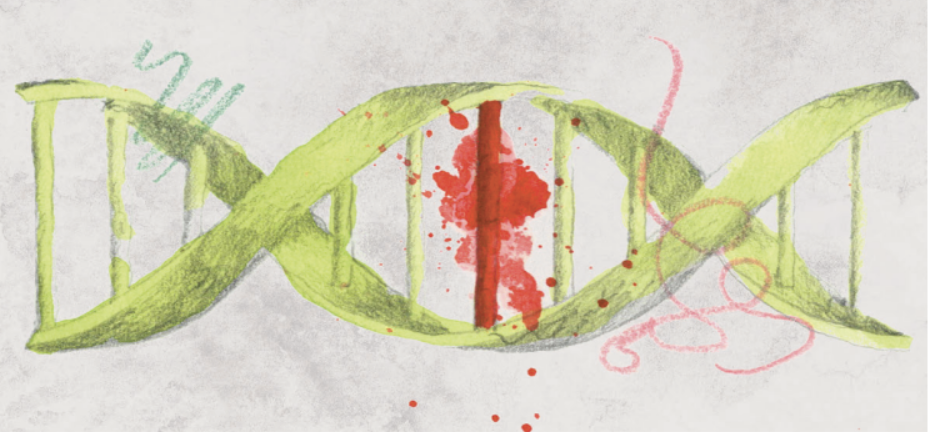
Clearly, the study of somatic mutations has great potential for improving our understanding of the brain’s abilities and dysfunction. There are many fascinating implications for this work, and researchers have already taken the first steps in determining the effects of these mutations on human individuality. It is exciting to know that we are more than just our inherited genetics and lifetime experiences—the mosaic-like nature of the brain adds a new layer of uniqueness and mutability to one’s identity.
References
- Bae, T. & Tomasini, L. (2017). Different mutational rates and mechanisms in human cells at pregastrulation and neurogenesis. Science , 359(6375): 550-555. doi:10.1126/science.aan8690.
- Lodato, M.A., Rodin, R. E., Bohrson, C. L., Coulter, M.E., Barton, A. R., & Kwon, M. (2017). Aging and neurodegeneration are associated with increased mutations in single human neurons. Science, 359(6375): 555-559. doi:10.1126/science.aao4426.
- 3 . Frank, S. A. (2010). Somatic evolutionary genomics: Mutations during development cause highly variable genetic mosaicism with risk of cancer and neurodegeneration. Natl. Acad. Sc i., 107(suppl 1): 1725–1730. doi:10.1073/pnas.0909343106.
- Jang, K. L. & Livesly, J. W. (1996). Heritability of the Big Five Personality Dimensions and Their Facets: A Twin Study. Journal of Personality , 64(3): 577-592. doi:10.1111/j.1467-6494.1996.tb00522.x.
- Stelmack, R. M. (1991). Advances in Personality Theory and Research. Journal of Psychiatry and Neuroscience , 16(3): 131-138.
- Kaminsky, Z., & Petronis, A. (2008). Epigenetics of personality traits: an illustrative study of identical twins discordant for risk-taking behavior. Twin Research and Human Genetics , 11(1): 1-11. doi:10.1375/twin.11.1.1.
- Nakashima, M., Saitsu, H., Takei, N., Tohyama, J., Kato, M., & Kitaura, H. (2015). Somatic mutations in the MTOR gene cause focal cortical dysplasia type IIb. Annals of Neurology , 78(3): 375–386. doi: 10.1002/ana.24444.
- Lim, J. S., Kim, W., Kang, H. C., Kim, S.H., Park, A. H., Park, E. K., Cho, Y. W., Kim, S., Kim, H. M., Kim, J. A., Kim, J., Rhee, H., Kang, S. G., Kim, H. D., Kim, D., Kim, D. S., & Lee, J. H. (2015). Brain somatic mutations in MTOR cause focal cortical dysplasia type II leading to intractable epilepsy. Nature Medicine, 21(4): 395-400. doi: 10.1038/nm.3824.
- Lee, J. H. (2016). Somatic mutations in disorders with disrupted brain connectivity. Experimental and Molecular Medicine , (48):e239. doi:10.1038/emm.2016.53.
- Marsh, A. A., & Finger, E. C. (2008). Reduced Amygdala Response to Fearful Expressions in Children and Adolescents With Callous-Unemotional Traits and Disruptive Behavior Disorders. The American Journal of Psychiatry , 165(6):712-720. doi:10.1176/appi.ajp.2007.07071145.