It was the summer of 1799. World famous chemist Sir Humphry Davy switched on his mercurial breathing machine, filling two bags with pure nitric oxide gas [1]. He exhaled deeply and brought one bag to his lips. Today was going to be a tremendous day for science. Davy took a deep breath, allowing the bag’s contents to enter his lungs. But as the nitric oxide trickled past his mouth, he choked and sputtered from the sour taste. He immediately released his lips from the bag and gasped for air, only to find that the incoming air burned him even more. The tiny amount of nitric oxide gas left in his mouth had instantly transformed into corrosive nitric acid. Davy suffered severe chemical burns to his tongue, throat, and teeth. He would later describe the taste of nitric oxide as “astringent and highly disagreeable,” and vowed to never again attempt such a rash experiment [1].
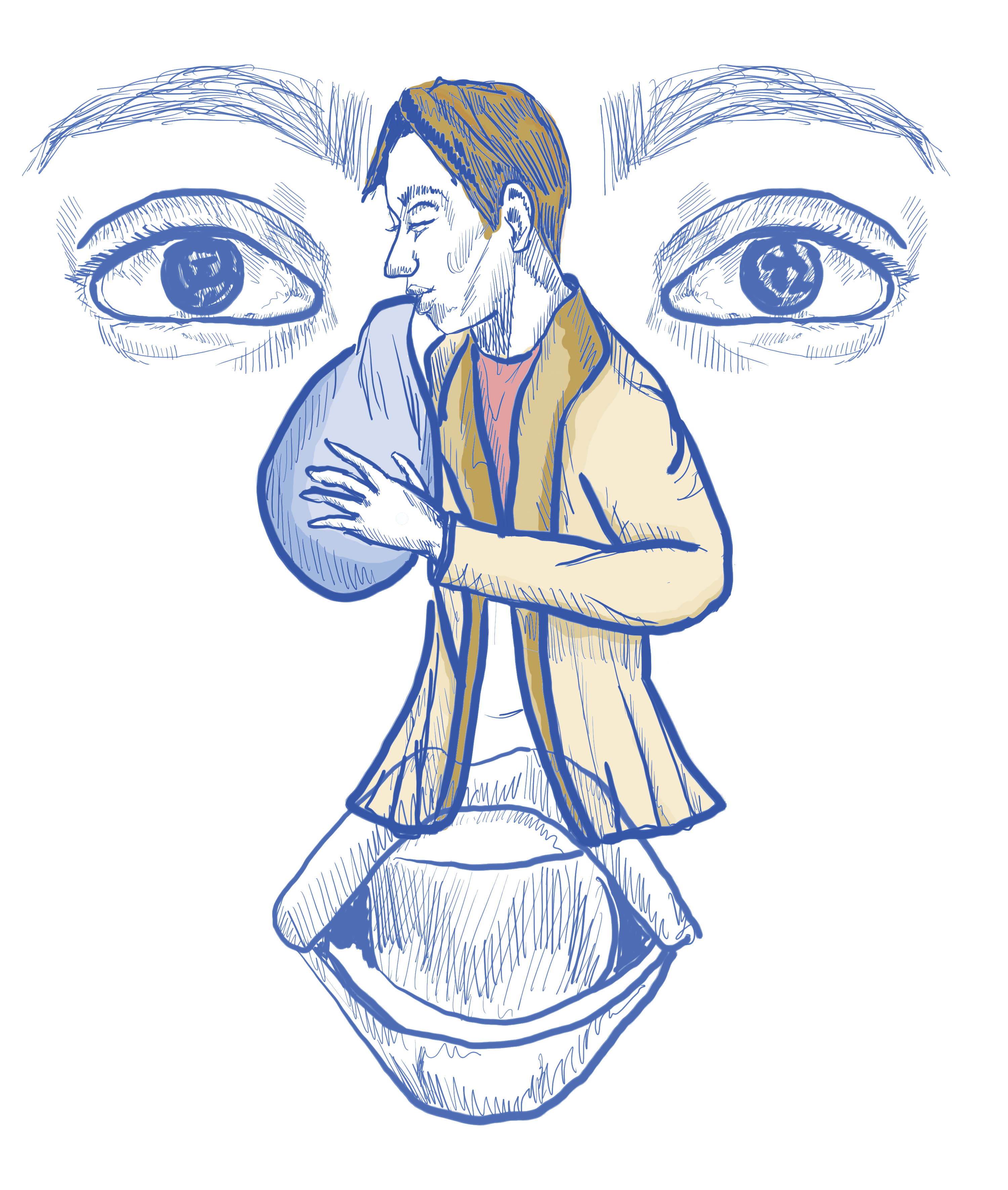
Despite how dangerous even a small amount of nitric oxide can be, the human body actually produces the gas endogenously. This surprising revelation shocked the field of physiology in the late 1980s, when researchers first discovered that naturally generated nitric oxide signals mediate human blood pressure [2] . This discovery alone earned the team a Nobel Prize in 1998, but research into this highly unusual biological chemical did not stop there [3] . In addition to its role in blood pressure, researchers discovered that nitric oxide signaling also controls yawning, erections, and even vision [4][5] . One topic that immediately stood out was the fact that nitric oxide signals exist in the human brain, and this spurred on decades of studies to uncover its purpose there [6] . In the years since then, it has become increasingly clear that nitric oxide plays multiple competing roles in signaling the formation of new memories, and the gas’s unorthodox properties may help explain these roles in multiple brain structures.
What Makes Nitric Oxide Special?
Before exploring what nitric oxide does in the brain, it is important to understand what exactly makes the gas so different from every other signaling molecule in the body. Nitric oxide (chemical formula NO) should not be confused with nitrous oxide (chemical formula N 2 O), which is better known as “laughing gas.” In stark contrast to his battle with nitric oxide, Sir Humphry Davy actually found nitrous oxide quite pleasant, and often threw lavish parties where he and his guests would inhale N 2 O recreationally [1] .
Perhaps the most obvious difference between nitric oxide and traditional chemical signals, or neurotransmitters, is that NO is a so-called “free radical” compound [7] . Chemically, this means that nitric oxide reacts very quickly with just about anything it touches, often producing even more reactive radicals in the process [7] . These free radicals can be harmful to cells, and the body maintains a robust system of antioxidants in order to prevent them from wreaking havoc [8] . It might at first appear strange that cells would deliberately produce something so harmful, but this property itself may provide benefits that outweigh the costs.
For instance, this extreme reactivity leads nitric oxide to react with a wide array of different molecular targets [9] . While traditional neurotransmitters are generally selective for a particular class of receptors, nitric oxide can directly react with many different proteins in many different ways. Nitric oxide’s most common target, a protein known as soluble guanylyl cyclase, is similarly diverse in its number of downstream targets [9] .
The unique chemical properties of nitric oxide do not stop there. The fact that nitric oxide is so small enables it to pass through cell membranes unhindered, which allows its broad range of potential targets to become even broader [7] . In the classical model of neurotransmission, cells package their signals for specific destinations, and the chemicals themselves are not able to deviate from the path. Neuron A releases neurotransmitter strictly onto the surface of Neuron B, which releases neurotransmitter only onto Neuron C, and so on.
But nitric oxide does not have the same limitations. As soon as NO is created, it can travel anywhere, dictated only by the concentration of the gas at a given point. Nitric oxide could go from Neuron A to Neuron B, or it could jump straight to Neuron C. In fact, it could even go backwards from Neuron B to Neuron A, or stay entirely within Neuron B to interact with itself. Because NO is so highly reactive, it is limited only by the short distance it can travel before it inevitably reacts with something. In practice this range can be surprisingly long, and greater activation increases the distance of the signal [10] .
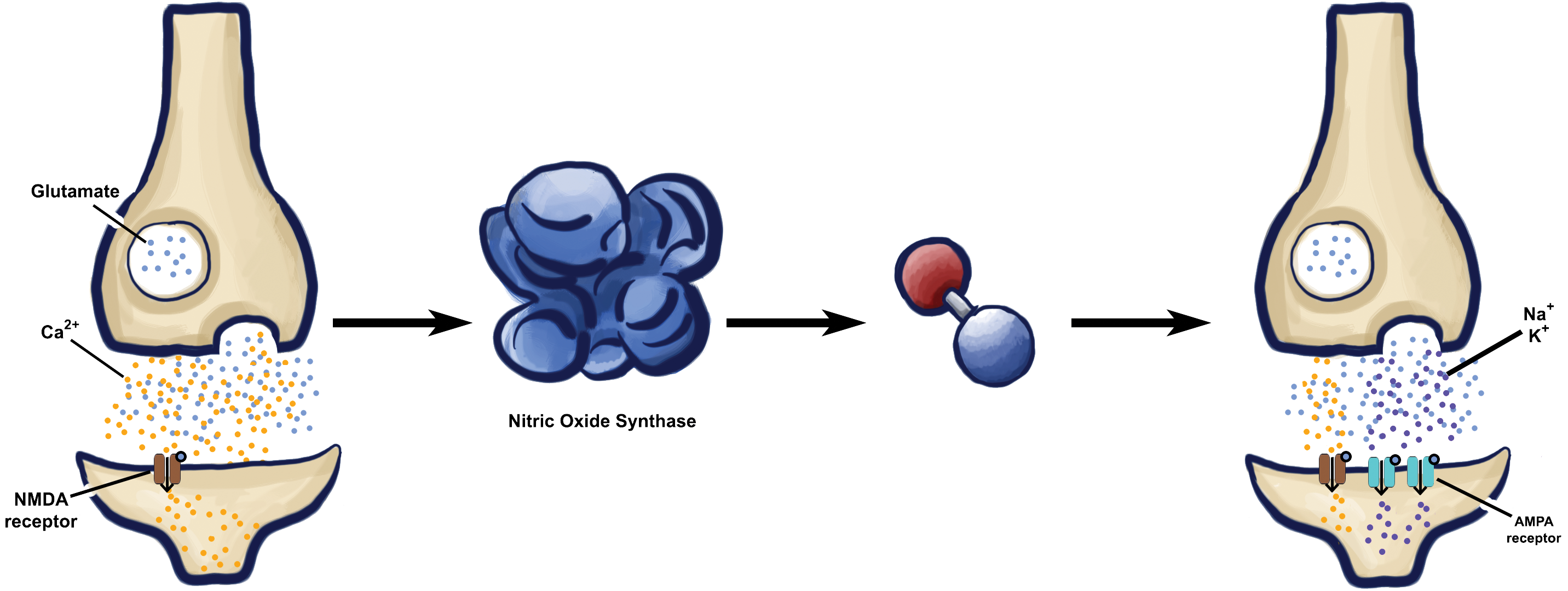
What This Means for Memory
This unique communication strategy makes nitric oxide an attractive candidate for researchers to explore retrograde signaling, or the process of messages traveling backwards. This capacity for retrograde signaling particularly drew researchers to study how nitric oxide might be involved with the formation of new memories. To understand why this is the case, it is first important to understand how memory actually works. On the cellular level, memory is typically the result of changes in the strength of the connection between two neurons over time [11] . This process is known as synaptic plasticity, and it is one of the most basic substrates for learning [11] .
Imagine Neuron A only barely connects with Neuron B before firing, but after repeated stimulation, the connection becomes much stronger. This change in synaptic efficacy encodes new information, which the brain incorporates into the storage of new memories. One of the easiest ways to change the efficacy of a synapse is to change how one neuron fires onto another by altering some property of the first neuron. This particular process is known as presynaptic plasticity, because it affects the presynaptic neuron [11] . Say Neuron A fires onto Neuron B, which then releases a retrograde signal back to Neuron A. This signal changes Neuron A in such a way as to increase its connection with Neuron B, ultimately increasing the efficacy of the synapse. Retrograde signaling is one possible way for cells to enact this type of synaptic change [11] .
NO and Classical Conditioning
In practice, nitric oxide signaling comprises both retrograde signaling and direct signaling, and both have been found to be important in the formation of new memories [11] . One type of memory that has been extensively linked with both aspects of nitric oxide signaling is classical conditioning. In its most basic form, classical conditioning involves the pairing of one novel stimulus with another stimulus that already evokes an innate response. When Pavlov trained his dogs to salivate to the sound of a dinner bell, he was teaching them through classical conditioning [12] . Numerous studies in species ranging from honeybees to humans have shown the importance of nitric oxide in classical conditioning models [13] . This holds especially true for olfactory classical conditioning, or the ability for animals to associate particular smells. For example, disrupting nitric oxide function in the region of the brain associated with scent at a critical time prevents sheep from being able to learn how to recognize their newborn babies [14] .
While nitric oxide has been almost universally implicated in different classical conditioning paradigms, that does not mean it always behaves the same way. For instance, removing nitric oxide either genetically or with NO-blocking drugs has been shown to impair some species’ ability to learn certain conditioned associations [15][16][17] . At the same time, other species have shown the exact opposite effect, suggesting that NO actually inhibits conditioning in these systems [18] . Even studies in the same species and same conditioning paradigm have shown differential effects on learning depending on the nature of the stimulus, or even the time of day [19][20] .
To explain this apparent disparity, it is important to understand that memory is not a singular process, and that different systems can have very different ways of encoding the same molecular information [21] . While it is generally accepted that the molecular basis of memory remains the same between species, nitric oxide’s versatility means it can play more than one role at the same time, leading to major variations in the way different systems make use of it [22][23] .
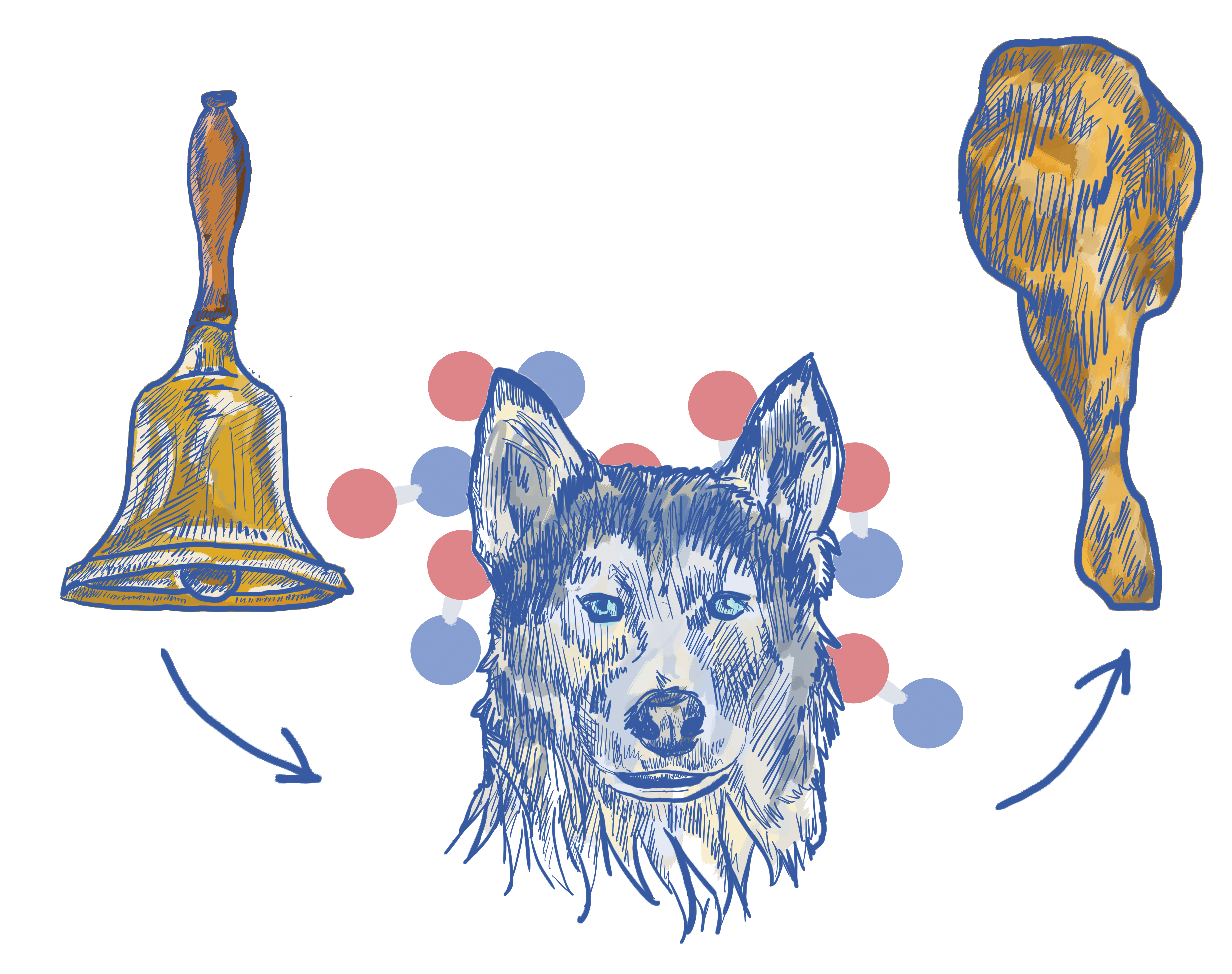
Other Types of Learning
Nitric oxide’s complicated role in classical conditioning carries through to other learning paradigms as well. Experiments in rodents in an area of the brain known as the hippocampus have shown a similarly important, albeit disparate role for nitric oxide. The hippocampus is the area of the brain that is most commonly associated with declarative memories, or memories of facts and events [24] . When most people think about memory, this is the kind they mean. Just like in circuits associated with classical conditioning, the hippocampus may need nitric oxide for some important types of plasticity [25] . Experiments in rodents that lack nitric oxide either genetically or due to NO-blocking drugs have demonstrated an impaired performance in water maze tasks, which require the animal to remember places it has been before [26][27] . Without NO, these animals fail to form spatial memories. Despite these observations, some research has also shown that increased nitric oxide production might actually be associated with hippocampal memory loss. For instance, rodents with overactive thyroids have impaired performance in water mazes, and also have much higher concentrations of NO in the hippocampus [28] .
NO and Memory-Related Disease
The thought that NO signaling might contribute to disease-related memory loss does not stop there. Recent research on Alzheimer’s disease has revealed some critical stages where nitric oxide signaling might play a role. In particular, haywire nitric oxide signaling has been proposed as one possible step in the degeneration of brain cells seen in Alzheimer’s patients [29] . At some point during the disease process, the brain selectively increases production of nitric oxide in order to mitigate further memory loss [30] . But because NO is a free radical, it eventually starts to degrade the cellular machinery in these parts of the brain. A major portion of the damage to critical memory structures seen in Alzheimer’s disease might occur through this mechanism [30] . At the same time, however, other aspects of nitric oxide signaling have been suggested to protect Alzheimer’s patients from further damage, making the situation extremely complicated [31] .
NO signaling may play a similarly duplicitous role in the loss of memory function with epilepsy, stroke, and even old age [32][33][34] . As we age, some of our learning and memory systems begin to erode. One popular hypothesis for this loss centers around sustained damage from free radicals [35] . At the same time, nitric oxide signaling changes as we age. Some parts of the brain increase their NO production, and others decrease it [36] . In aging rodents, there have been successful experiments in attempting to restore declarative memory function by replenishing lost nitric oxide production [37] . Rodents that would otherwise perform worse with age at water maze tasks may salvage some of that function with added NO to the hippocampus [37] . But once again, this is not the end of the story. Nitric oxide is still a free radical, and the accumulated damage from NO and its products may still have many negative effects on memory function with age [38] . Furthermore, its role in controlling blood vessels may intersect with its role in the brain itself, since disrupting blood flow can also explain changes in neural function [39] . This may also ultimately explain some of NO’s involvement in Alzheimer’s pathology.
Researchers continue to explore the complex and often double-edged role of nitric oxide in learning and memory systems. While there are no current drugs on the market that take advantage of nitric oxide pathways for the treatment of memory dysfunction in humans, there have been a wealth of animal studies exploring how NO could be used to help treat disease. A number of extremely successful drugs already exploit nitric oxide pathways, including the erectile dysfunction drug Viagra, which prevents the degradation of NO’s most immediate downstream signal. In line with the idea that NO signaling is vital for proper memory function, Viagra has been shown to stave off memory loss in Alzheimer’s disease rodent models, and this effect is not limited to enhanced cerebral blood flow [41] . In fact, recent human clinical trials have explored whether Viagra analogs might treat certain types of dementia, although the results remain unpublished [41] . Putting this all together, it is clear that nitric oxide’s role in learning and memory has broad implications for both normal function and disease. While it can be difficult to balance the wildly different effects of nitric oxide under different conditions, researchers continue to build a better understanding of the molecule in many neural circuits. This understanding might someday help fight against memory-related disease, although there is still a long way to go.
- Sprigge, J. S. (2002). Sir Humphry Davy; his researches in respiratory physiology and his debt to Antoine Lavoisier. Anaesthesia , 57 (4), 357-364.
- Ignarro, L. J., Buga, G. M., Wood, K. S., Byrns, R. E., & Chaudhuri, G. (1987). Endothelium-derived relaxing factor produced and released from artery and vein is nitric oxide. Proceedings of the National Academy of Sciences , 84 (24), 9265-9269
- SoRelle, R. (1998). Nobel prize awarded to scientists for nitric oxide discoveries. Circulation . 1998 Dec 1;98(22):2365-6.
- Melis, M. R., & Argiolas, A. (1997). Role op central nitric oxide in the control of penile erection and yawning. Progress in Neuro-Psychopharmacology and Biological Psychiatry, 21(6), 899-922.
- Cudeiro, J., & Rivadulla, C. (1999). Sight and insight–on the physiological role of nitric oxide in the visual system. Trends in neurosciences, 22(3), 109-116.
- Garthwaite, J., Charles, S. L., & Chess-Williams, R. (1988). Endothelium-derived relaxing factor release on activation of NMDA receptors suggests role as intercellular messenger in the brain. Nature, 336(6197), 385.
- Lancaster Jr, J. R. (2015). Nitric oxide: a brief overview of chemical and physical properties relevant to therapeutic applications. Future science OA , 1 (1).
- Packer, L., & Prilipko, L. (Eds.). (2012). Free radicals in the brain: aging, neurological and mental disorders . Springer Science & Business Media.
- Lane, Paul B., and Steven S. Gross. “Nitric oxide: promiscuous and duplicitous.” Science and Medicine 8, no. 2 (2001): 96-107.
- Lancaster Jr, J. R. (1997). A tutorial on the diffusibility and reactivity of free nitric oxide. Nitric Oxide , 1 (1), 18-30.
- Yang, Y., & Calakos, N. (2013). Presynaptic long-term plasticity. Frontiers in synaptic neuroscience , 5 , 8.
- Jarius, S., & Wildemann, B. (2015). And Pavlov still rings a bell: summarising the evidence for the use of a bell in Pavlov’s iconic experiments on classical conditioning. Journal of neurology , 262 (9), 2177-2178.
- Hawkins, R. D. (1996). NO honey, I don’t remember. Neuron , 16 (3), 465-467.
- Kendrick, K. M., Guevara-Guzman, R., Zorrilla, J., Hinton, M. R., Broad, K. D., Mimmack,
- A., & Ohkura, S. (1997). Formation of olfactory memories mediated by nitric oxide. Nature , 388 (6643), 670.
- Antonov, I., Ha, T., Antonova, I., Moroz, L. L., & Hawkins, R. D. (2007). Role of nitric oxide in classical conditioning of siphon withdrawal in Aplysia. Journal of Neuroscience, 27(41), 10993-11002.
- Jami, S. A., Wright, W. G., & Glanzman, D. L. (2007). Differential classical conditioning of the gill-withdrawal reflex in Aplysia recruits both NMDA receptor-dependent enhancement and NMDA receptor-dependent depression of the reflex. Journal of Neuroscience , 27 (12), 3064-3068.
- Yang, Q., Kuzyk, P., Antonov, I., Bostwick, C. J., Kohn, A. B., Moroz, L. L., & Hawkins, R.
- (2015). Hyperpolarization-activated, cyclic nucleotide-gated cation channels in Aplysia: contribution to classical conditioning. Proceedings of the National Academy of Sciences , 112 (52), 16030-16035.
- Du, W., Weiss, H., & Harvey, J. A. (2000). Associative learning is enhanced by selective neuronal nitric oxide synthase inhibitors and retarded by a nitric oxide donor in the rabbit. Psychopharmacology , 150 (3), 264-271.
- Yabumoto, T., Takanashi, F., Kirino, Y., & Watanabe, S. (2008). Nitric oxide is involved in appetitive but not aversive olfactory learning in the land mollusk Limax valentianus. Learning & Memory , 15 (4), 229-232.
- Gage, S. L., & Nighorn, A. (2014). The role of nitric oxide in memory is modulated by diurnal time. Frontiers in systems neuroscience , 8 , 59.
- Kim, J. J., & Baxter, M. G. (2001). Multiple brain-memory systems: the whole does not equal the sum of its parts. Trends in neurosciences , 24 (6), 324-330.
- Ancient rules of memory. The molecules and mechanisms of memory evolved long before their ‘modern’ use in the brain
- Susswein, A. J., Katzoff, A., Miller, N., & Hurwitz, I. (2004). Nitric oxide and memory. The Neuroscientist , 10 (2), 153-162.
- Eichenbaum, H., & Cohen, N. J. (2014). Can we reconcile the declarative memory and spatial navigation views on hippocampal function?. Neuron , 83 (4), 764-770.
- Harooni, H. E., Naghdi, N., Sepehri, H., & Rohani, A. H. (2009). The role of hippocampal nitric oxide (NO) on learning and immediate, short-and long-term memory retrieval in inhibitory avoidance task in male adult rats. Behavioural brain research , 201 (1), 166-172.
- Weitzdoerfer, R., Hoeger, H., Engidawork, E., Engelmann, M., Singewald, N., Lubec, G., & Lubec, B. (2004). Neuronal nitric oxide synthase knock-out mice show impaired cognitive performance. Nitric oxide , 10 (3), 130-140.
- Qiang, M., Chen, Y., Wang, R., Wu, F. M., & Qiao, J. T. (1997). Nitric oxide is involved in the formation of learning and memory in rats: studies using passive avoidance response and Morris water maze task. Behavioural pharmacology .
- Hosseini, M., Dastghaib, S. S., Rafatpanah, H., Hadjzadeh, M. A. R., Nahrevanian, H., & Farrokhi, I. (2010). Nitric oxide contributes to learning and memory deficits observed in hypothyroid rats during neonatal and juvenile growth. Clinics , 65 (11), 1175-1181.
- Aliev, G., Palacios, H. H., Lipsitt, A. E., Fischbach, K., Lamb, B. T., Obrenovich, M. E., … & Bragin, V. (2009). Nitric oxide as an initiator of brain lesions during the development of Alzheimer disease. Neurotoxicity research , 16 (3), 293-305.
- Balez, R., & Ooi, L. (2016). Getting to NO Alzheimer’s disease: neuroprotection versus neurotoxicity mediated by nitric oxide. Oxidative medicine and cellular longevity , 2016 .
- Chakroborty, S., Kim, J., Schneider, C., West, A. R., & Stutzmann, G. E. (2015). Nitric oxide signaling is recruited as a compensatory mechanism for sustaining synaptic plasticity in Alzheimer’s disease mice. Journal of Neuroscience , 35 (17), 6893-6902.
- Banach, M., Piskorska, B., J Czuczwar, S., & K Borowicz, K. (2011). Nitric oxide, epileptic seizures, and action of antiepileptic drugs. CNS & Neurological Disorders-Drug Targets (Formerly Current Drug Targets-CNS & Neurological Disorders) , 10 (7), 808-819.
- Chen, Z. Q., Mou, R. T., Feng, D. X., Wang, Z., & Chen, G. (2017). The role of nitric oxide in stroke. Medical gas research , 7 (3), 194.
- Domek-Łopacińska, K. U., & Strosznajder, J. B. (2010). Cyclic GMP and nitric oxide synthase in aging and Alzheimer’s disease. Molecular neurobiology , 41 (2-3), 129-137.
- Packer, L., & Prilipko, L. (Eds.). (2012). Free radicals in the brain: aging, neurological and mental disorders . Springer Science & Business Media.
- Ledo, A., Lourenço, C. F., Caetano, M., Barbosa, R. M., & Laranjinha, J. (2015). Age-associated changes of nitric oxide concentration dynamics in the central nervous system of fisher 344 rats. Cellular and molecular neurobiology , 35 (1), 33-44.
- Pitsikas, N., Rigamonti, A. E., Cella, S. G., Sakellaridis, N., & Muller, E. E. (2005). The nitric oxide donor molsidomine antagonizes age-related memory deficits in the rat. Neurobiology of aging , 26 (2), 259-264.
- Steinert, J. R., Chernova, T., & Forsythe, I. D. (2010). Nitric oxide signaling in brain function, dysfunction, and dementia. The Neuroscientist , 16 (4), 435-452.
- Grinberg, L. T., & Thal, D. R. (2010). Vascular pathology in the aged human brain. Acta neuropathologica , 119 (3), 277-290.
- Puzzo, D., Staniszewski, A., Deng, S. X., Privitera, L., Leznik, E., Liu, S., … & Arancio, O. (2009). Phosphodiesterase 5 inhibition improves synaptic function, memory, and amyloid-β load in an Alzheimer’s disease mouse model. Journal of Neuroscience , 29 (25), 8075-8086.
- Pauls, M. M., Clarke, N., Trippier, S., Betteridge, S., Howe, F. A., Khan, U. & Rolfe, D. (2017). Perfusion by Arterial Spin labelling following Single dose Tadalafil In Small vessel disease (PASTIS): study protocol for a randomised controlled trial. Trials , 18 (1), 229.
- Lane, P. B., & Gross, S. S. (2001). Nitric oxide: promiscuous and duplicitous. Science and Medicine , 8 (2), 96-107.