Despite there being a large unmet need for treatments in neurological disorders, big pharma is beginning to withdraw from drug development due to the challenge of crossing the blood-brain barrier, while small pharma is continuing to take the risk.
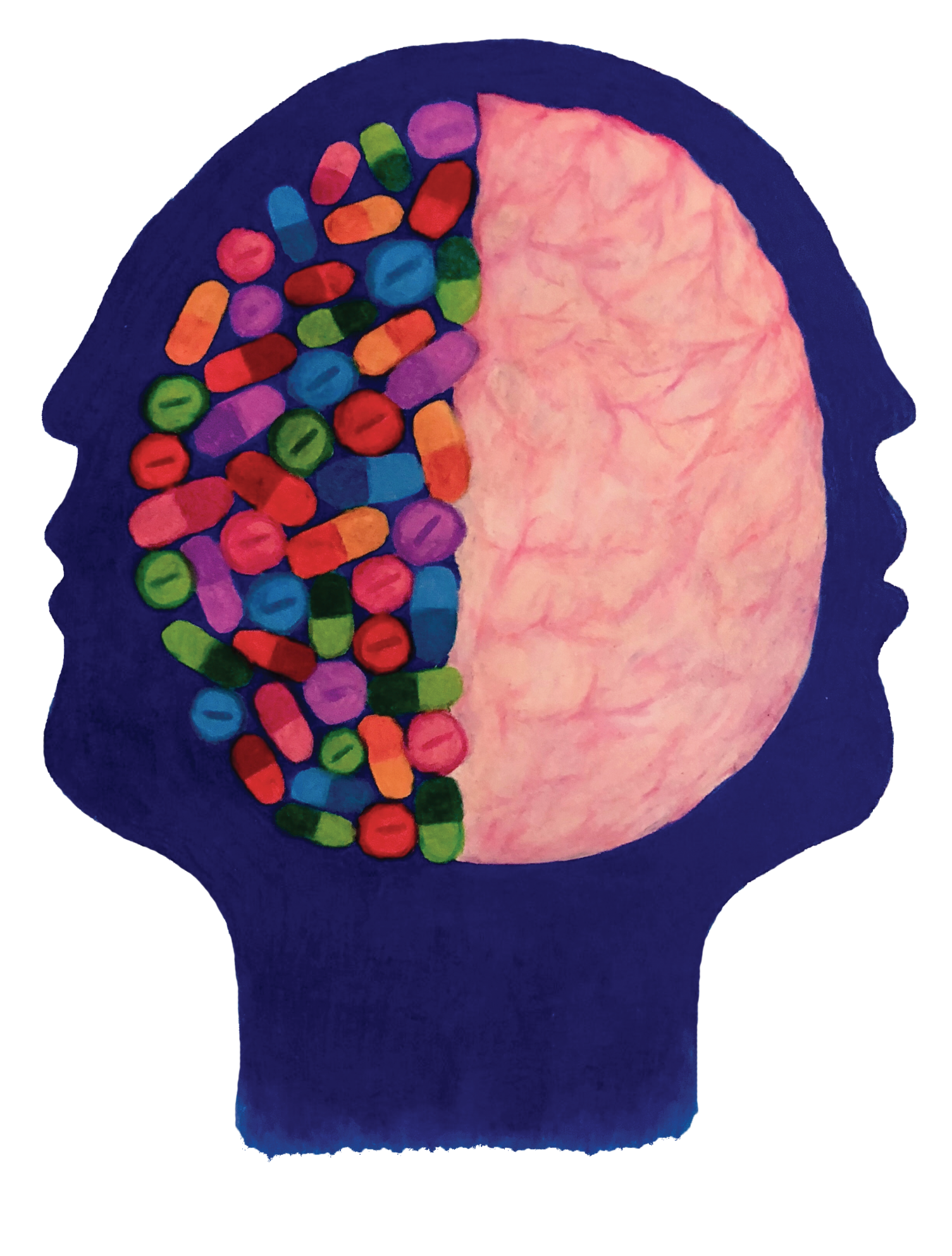
Often, when people experience symptoms that interfere with their lives, they have the choice to alleviate those symptoms. If you have a bacterial infection, what do you do? You can take antibiotics. If you have a sore throat, you take Tylenol. Headache? Advil. But what if you find that there are no options available for your symptoms? For people with minor and temporary issues like a cold, a lack of treatment options is solely an inconvenience. However, for those with long-term debilitating conditions, it is a different story. A lack of adequate treatment options is what many people with neurological disorders currently face.
Neurological disorders affect one billion people worldwide, account for 20% of deaths, and decrease the quality of life for the people directly and indirectly affected more than any other disorder. [1] For years, the pharmaceutical industry has been heavily invested in developing treatments for neurological disorders, especially Alzheimer’s, Parkinson’s and epilepsy. Although some treatments do exist, they are not effective for the majority of patients and offer only a short-term solution, as they address symptoms rather than the underlying causes. [2] The blood-brain barrier (BBB) has stopped most efforts by big pharma to develop new drugs for neurological disorders. After decades of unsuccessful drug development, big pharma is beginning to withdraw from this treatment field, depleting the availability of resources and threatening the hope for future medications.
The Challenges
Drug development is a challenging field; drugs must enter the bloodstream, reach their target organ, and have a specific effect there. Different forms of drug administration pose different levels of challenges. Injections go straight into the bloodstream, while the more user-friendly oral administration requires the drug to be absorbed into the blood through the intestines. Once at the target organ, there are three ways a molecule can pass into the organ: diffusion through the cells, diffusion between the cells, and active transport. All three pathways impose strict limits on, among other things, the hydrophilicity, charge, structure, and size of the molecules. [3] Other considerations are circulation time in the body and side effects on other organs. These issues are present in most forms of drug delivery, and the industry has developed ways to overcome them.
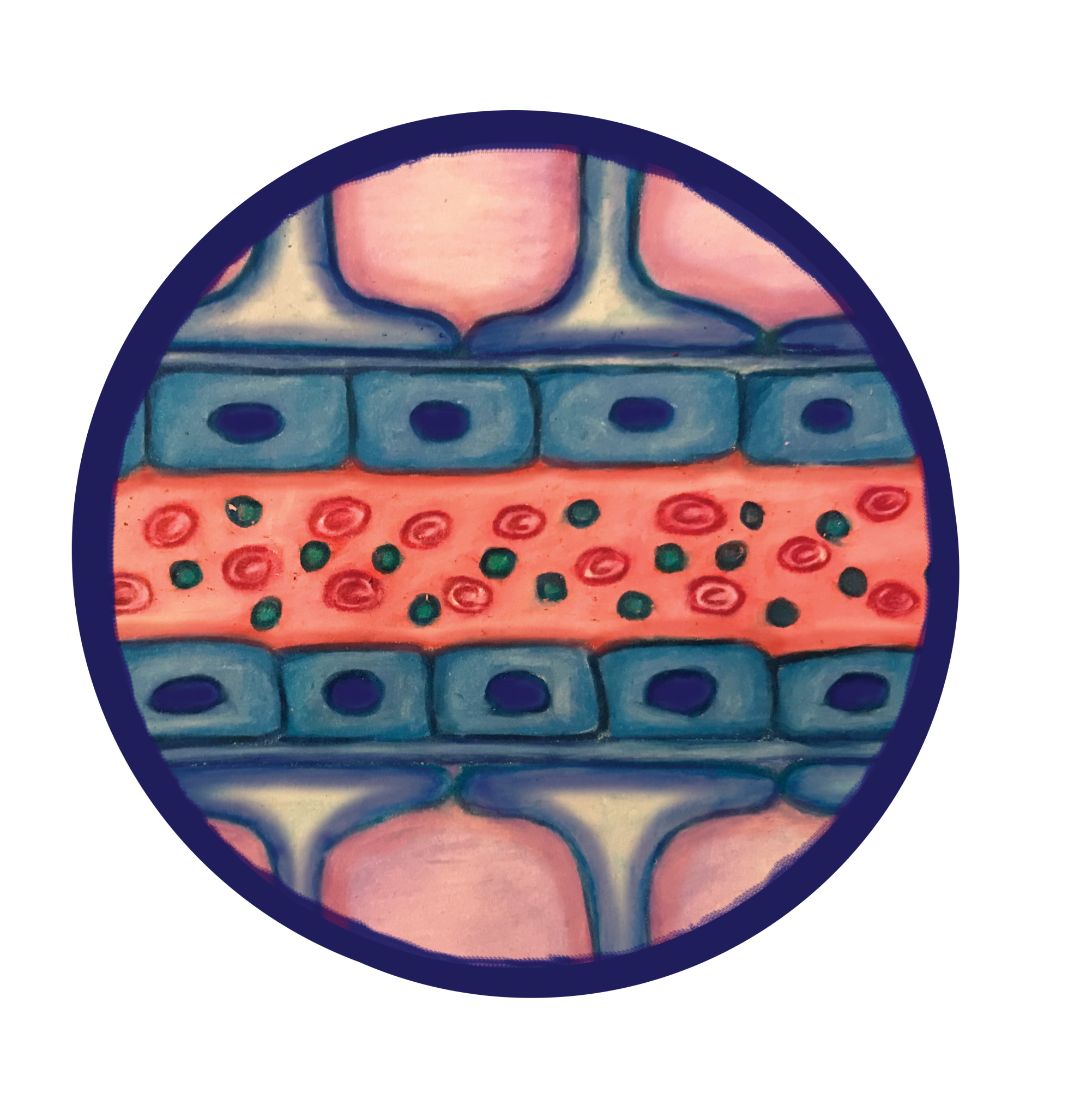
For neurological disorders, there is an additional challenge that the pharmaceutical industry has yet to overcome: the BBB. Nearly all large drug molecules and 98% of small molecules cannot pass the BBB. [4] In comparison to the one-layer epithelium in other organs, the BBB has a double-layer for extra protection. [5] Around the capillaries in the brain, there is a layer of epithelial cells, and on top of the epithelial cells exists another layer of cells known as astrocytes. [6] Additionally, both layers of the BBB have tight junctions, intracellular adhesions that do not allow molecules to pass between the cells, whereas most other organs have leaky junctions, characterized by larger gaps and increased permeability between cells due to fewer adhesive molecules. [5] Furthermore, even if the drug were to enter the cells or the tight junctions, both the epithelial cells and astrocytes are equipped with a larger amount and variety of efflux pumps than any other epithelial layer in the body. [7] These pumps recognize a wide range of molecules and export them back into the bloodstream. This protection system only allows small molecules with specific chemical properties to pass into the brain.
The extreme limitations on molecular structure and size imposed by the BBB create a trade-off that has been difficult to balance thus far. In order to design an effective drug, companies first need to create a molecule that they hypothesize will have the desired effect on neurons when it enters the brain. Additionally, there are requirements for the drug to make it into the bloodstream and to the brain. These properties are often contradictory to those needed to cross the BBB. [3]
The Demoralization of Big Pharma
Neurological drugs have one of the longest development phases and lowest success rates in the drug development industry. [8] The last major breakthrough in the industry was the development of a treatment for Parkinson’s disease in the 1960s. [9] The drug levodopa has miraculous effects for early Parkinson’s, alleviating symptoms completely; however, it is only temporarily effective, as it does not stop the progression of the disease. [9] Over the past 60 years the quest for alternative therapies has stagnated; there is still no drug to treat the underlying cause of Parkinson’s. Similarly, within the last 40 years, antidepressants have remained the same, and the number of treatment-resistant epilepsy cases has not changed. Additionally, more than 99.6% of human trials for Alzheimer’s drugs have failed over the last 20 years. [10]
It is unclear whether trials are failing due to a lack of understanding of the diseases or whether the BBB is preventing drug entry, since animal models differ largely from humans in both respects. [11] Failure can likely be attributed to a combination of ineffective pathway targeting and dose limitations created by the BBB. Over 99% of company investment in neurology is devoted to discovering new active drug compounds, and less than 1% goes toward development of drug delivery to the brain. [4] Multiple different pathways have been targeted by novel drug molecules, but a common problem in many trials is the BBB, suggesting that the BBB is the main reason for trial failures. After losing billions of dollars, big pharma is re-evaluating the risks and benefits of investing in neurological drug development.
Following proposed drug failures in 2015, there was a 50% decrease in funding allocated to neurology research in 2016. [12, 13] In 2016, the amount of companies pursuing novel treatments was the lowest of the decade with a decrease of 13% from the 10-year average. [12] In January of 2018, Pfizer, one of the highest grossing pharmaceutical companies in the world, shut down their neuro-investigation department following the failure of one of their human trials for an Alzheimer’s treatment. [14, 15, 16] Pfizer has invested in treatments for neurological diseases for decades, losing billions of dollars. Following previous drug designs that went into clinical trials and failed, Pfizer was hopeful their new design would succeed. Its failure was the last straw. Similar disappointments have led to the recent downsizing of the neurological research departments at other big pharmaceutical companies such as Eli Lilly, Axovant, AstraZeneca, and GlaxoSmithKline. [13]
The cost of drug development is similar in other departments. However, the low success rate in neurological drug development has turned the calculated risk for profit into an almost definite loss. Getting a drug or method of administration from the lab to the clinic costs an estimated $800 million per drug and takes approximately ten years. [17] Drugs go through three phases of clinical trials before a claim can be filed for FDA approval. These trials and FDA approval take around ten years to complete, while the patent the companies own is only active for six years. [18] After that, the intellectual property becomes public, meaning that anyone can access the design and create a cheaper, generic version, taking most of big pharma’s profit. The top grossing drug in 2017 brought in around $15 billion, making it worth 110 clinical trials within the six-year patent expiration time. [19] It is unreasonable for companies to make such a large investment into a high-risk field when they can also invest in low-risk fields.
Innovation in Small Pharma
While big pharma is largely leaving the neurological drug industry, some small start-ups are still
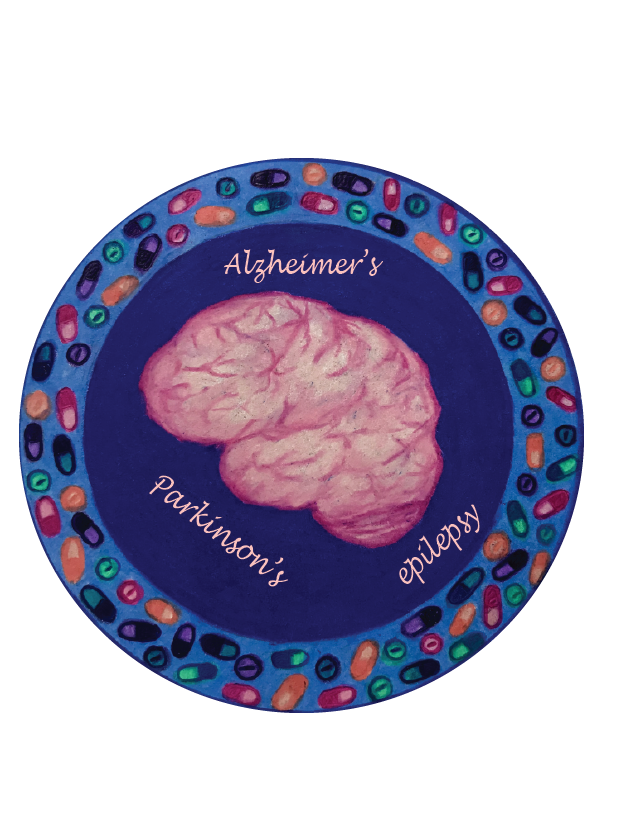
committed to the field and are taking the calculated risk. Big pharma refers to large companies with over 500 employees and a budget of over one billion dollars, whereas small pharma is defined as new companies stemming from a single idea with a small budget. In contrast to big pharma, these companies usually commit to one idea due to limited resources. The company founders have confidence that their approach may break the 60-year neurological drug development slump.
One such company is Impel NeuroPharma, which is pursuing a novel form of drug delivery: intranasal. [20] The upper nasal cavity, targeted by Impel NeuroPharma’s nasal spray, has a connection to two brain nerves, the olfactory and trigeminal nerves. [21] These nerves offer a direct connection to the brain, bypassing the BBB. Drug molecules are delivered through the nose and absorbed into the epithelium from which they travel extracellularly along the nerves. [22] This allows the drug to rapidly travel along the trigeminal and olfactory pathways that are widely spread throughout the brain and diffuse into the surrounding brain tissue. [22] Impel NeuroPharma patented their nasal delivery device in 2017 after the nasal spray was successful in human imaging and animal drug delivery trials. [23, 24] The company is currently using their device to conduct human trials with drugs for migraines, Alzheimer’s, and Parkinson’s. [20]
Other companies are working with nanoparticles, chemical coatings and methods of temporarily creating gaps in the BBB. [6] Permeabilizing the BBB is an idea that has been around since the 1980s and has still not been approved for treatment due to low efficacy and breach of the protective barrier, leading to a high risk of brain damage and infection. [6, 25]
Most of the small companies receive funding from big pharma once their original ideas show promising results in preclinical animal trials. Currently, big pharma funds 78% of all trials in neurological drug development, making it the most powerful force in the field. [10] The remaining trials are sponsored largely by investment companies, usually from the biotechnology industry, and partially by the government. [26] With the gradual exit of big pharma from neurological research and development, where does this leave the industry? Small pharma will continue to develop small ideas but will rarely be able to afford the clinical trials necessary for FDA approval. There is hope that with new developments from small pharma, big pharma will see an incentive to re-enter and re-invest in the field.
- Feigin, V.L., Abajoir, A.A., Abate, K.H., Abd-Allah, F., Abdulle, A.M., et al. (2017). Global, regional, and national burden of neurological disorders during 1990–2015: a systematic analysis for the Global Burden of Disease Study 2015. Lancet Neurology, 16(11) , 877-97. doi: 10.1016/ S1474-4422(17)30299-5.
- World Health Organization (2007). Neurological disorders a public health approach. Neurological Disorders: Public Health Challenges, chapter 3 , 41-94. ISBN: 978 92 4 156336 9.
- Banks, W. (2009). Characteristics of compounds that cross the blood-brain barrier. BMC Neurology, 9(Suppl 1) , S3. doi: 10.1186/1471-2377-9-s1-s3.
- Pardridge, W. (2009). Alzheimer’s disease drug development and the problem of the blood-brain barrier. Alzheimer’s & Dementia, 5(5) , 427-432. doi: 10.1016/j.jalz.2009.06.003.
- Fischbarg, J. (2010). Fluid Transport Across Leaky Epithelia: Central Role of the Tight Junction and Supporting Role of Aquaporins. Physiological Reviews, 90(4), 1271-1290. doi: 10.1152/physrev.00025.2009.
- Patel, M.M. & Patel, B.M. (2017). Crossing the Blood–Brain Barrier: Recent Advances in Drug Delivery to the Brain. CNS Drugs, 31(2) , 109-133. doi: 10.1007/s40263-016-0405-9.
- Sanchez-Covarrubias, L., Slosky, L., Thompson, B., Davis, T. & Ronaldson, P. (2014). Transporters at CNS Barrier Sites: Obstacles or Opportunities for Drug Delivery? Current Pharmaceutical Design, 20(10) , 1422-1449. doi: 10.1186/1471-2377-9-S1-S3 .
- BIO (2016). Clinical Development Success Rates 2006-2015. BIO Industry Analysis , 1-17.
- Cotzias, G.C., Van Woert, M.H. & Schiffer, L.M. (1967). Aromatic Amino Acids and Modification of Parkinsonism. The New England Journal of Medicine, 267 , 374-379. doi: 10.1056/NEJM196702162760703.
- Cummings, J.L., Morstorf, T. & Zhong, K. (2014). Alzheimer’s disease drug-development pipeline: few candidates, frequent failures. Alzheimer’s Research & Therapy, 6(37) , 1-7. doi: 10.1186/alzrt269.
- Pankevich, D., Altevogt, B., Dunlop, J., Gage, F. & Hyman, S. (2014). Improving and Accelerating Drug Development for Nervous System Disorders. Neuron , 84(3), 546-553. doi: 10.1016/j.neuron.2014.10.007.
- Thomas, D. & Wessel, C. (2016). Emerging Therapeutic Company Investment and Deal Trends. BIO Industry Analysis, 1-38.
- Tracy, H.M. (2016). The Neuro Funding Rollercoaster. Cerebrum . http://www.dana.org/Cerebrum/2016/The_Neuro_Funding_Roller_Coaster/.
- Dolsten, M. (2018). Learn More About Our Neuroscience R&D Decision. Pfizer Press release .
- Pfizer. (2017). 2017 Pfizer Annual Report to Shareholders. Annual Reports. https://investors.pfizer.com/financials/annual-reports/default.aspx.
- Vandenberghe, R., Rinne, J., Boada, M., Katayama, S., Scheltens, P., et al. (2016). Bapineuzumab for mild to moderate Alzheimer’s disease in two global, randomized, phase 3 trials. Alzheimer’s Research & Therapy, 8(18), 1-13. doi: 10.1186/s13195-016-0189-7.
- DiMasi, J., Hansen, R. & Grabowski, H. (2003). The price of innovation: new estimates of drug development costs. Journal of Health Economics, 22(2), 151-185. doi: 10.1016/s0167-6296(02)00126-1.
- Van Norman, G. (2016). Drugs, Devices, and the FDA: Part 1 An Overview of Approval Processes for Drugs. JACC: Basic to Translational Science, 1(3), 170-180. doi: 10.1016/j.jacbts.2016.03.002.
- Lindsley, C. (2017). New 2016 Data and Statistics for Global Pharmaceutical Products and Projections through 2017. ACS Chemical Neuroscience, 8(8), 1635-1636. doi: 10.1021/acschemneuro.7b00253.
- Hoekman, J.D., Hite, M., Brunelle, A., Relethford, J. & Ho, R.J.Y. (2017). US Grant No. US9550036B2. Washington, DC: U.S. Patent and Trademark Office.
- Ruigrok, M. & de Lange, E. (2015). Emerging Insights for Translational Pharmacokinetic and Pharmacokinetic-Pharmacodynamic Studies: Towards Prediction of Nose-to-Brain Transport in Humans. The AAPS Journal , 17(3) , 493-505. doi: 10.1208/s12248-015-9724-x.
- Chapman, C., Frey, W., Craft, S., Danielyan, L. & Hallschmid, M. (2012). Intranasal Treatment of Central Nervous System Dysfunction in Humans. Pharmaceutical Research, 30(10), 2475-2484. doi: 10.1007/s11095-012-0915-1.
- Krishnan, J., Arun, P., Appu, A., Vijayakumar, N. & Figueiredo. (2016). Intranasal delivery of obidoxime to the brain prevents mortality and CNS damage from organophosphate poisoning. NeuroToxicology, 53, 64-73. doi: 10.1016/j.neuro.2015.12.020.
- Hoekman, J., Brunelle, A., Hite, M., Kim, P. & Fuller, C. (2013). SPECT Imaging of Direct Nose-to-Brain Transfer of MAG-3 in Man. AAPS Poster , W4009.
- Neuwelt, E., Frenkel, E., Diehl, J., Vu, L. & Rapoport, S. (1980). Reversible osmotic blood-brain barrier disruption in humans: implications for the chemotherapy of malignant brain tumors. Neurosurgery, 7(1) , 44-52. doi: 10.1172/JCI109509.
- Moses, H., Matheson, D., Cairns-Smith, S., George, B., Palisch, C. & Dorsey, E. (2015). The Anatomy of Medical Research. JAMA, 313(2), 174. doi: 10.1001/jama.2014.15939.