With the new millennium, interconnectivity has undoubtedly become an integral part of society; and with it, the need to learn new information with ease and grace. Traditionally, this has meant years of school and specialized training within a field; however, as technology interfaces with the classroom, researchers have taken cues from sleep studies to better research the neurobiology of learning. Through these studies, they have found that neural oscillations play a significant role in the formation of memories and recall necessary for learning.
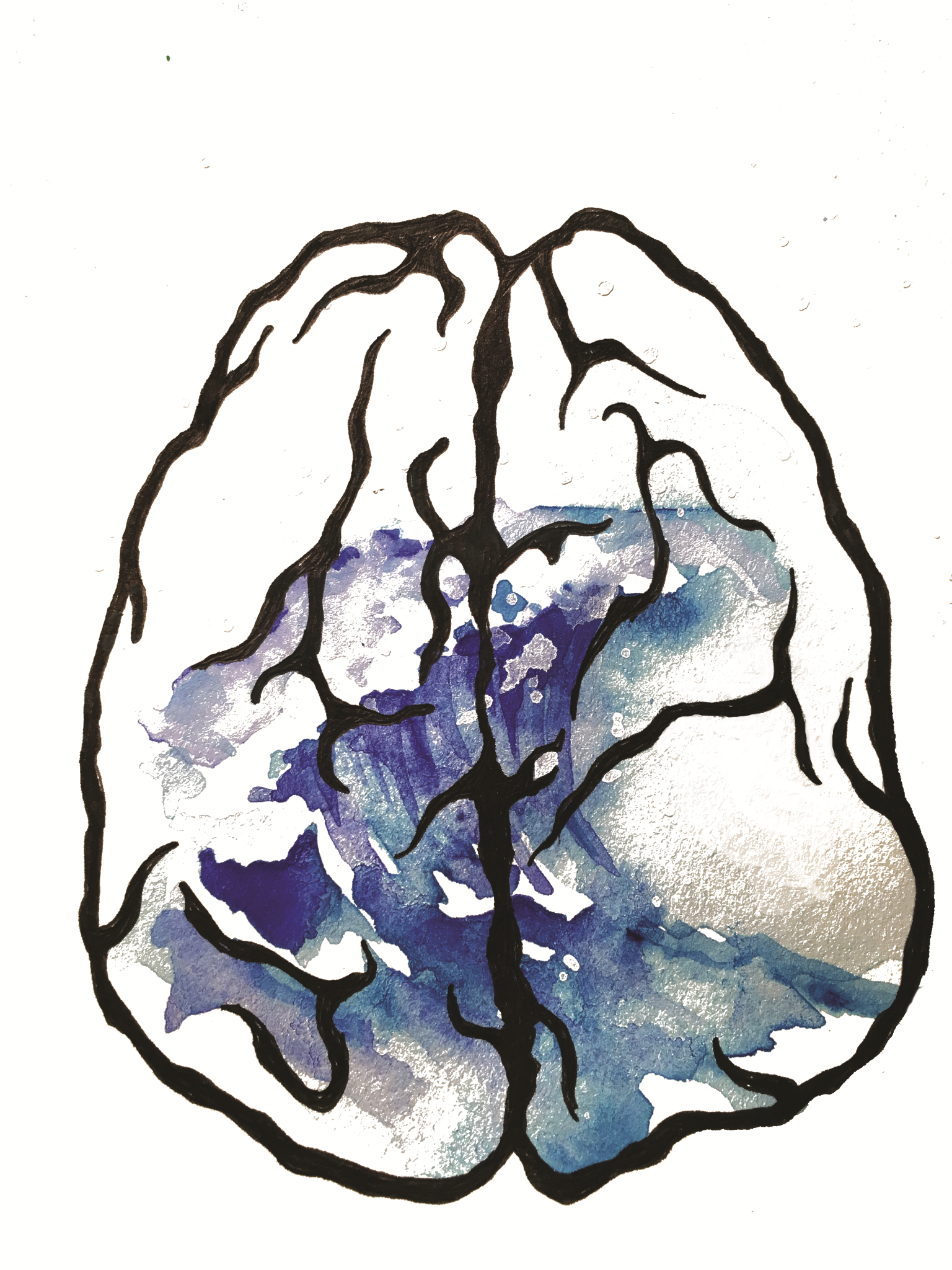
Oscillations and Waves
Neural oscillations, commonly known as brain waves, are the electrical patterns of action potentials that groups of neighboring neurons use to communicate with other clusters of neurons [1]. In order for this communication to occur, neurons conduct electricity individually but create a bigger wave of electrical activity together that is sent to other parts of the brain. These parts of the brain then send back their own waves, similar to how a phone call requires both a caller and receiver for a conversation to take place [1][2]. In the brain, this conversation allows neurons to send packets of information so other neurons know what to do next [1][2]. Electrical signals occur at different frequencies and add up to become a wave composed of many frequencies, resulting in a “neural oscillation" [3].
Varying amplitudes are directly affected by the number of neurons firing simultaneously, which equates to how much information is sent [1]. All of these waves come together to form a unique signal that tell the brain to perform a task, but they are never isolated in the brain. There are at least eight different kinds of oscillations, all of which serve different functions. These include alpha, beta, gamma, delta, theta, slow wave, slower wave, and below threshold waves. Though scientists are still researching how each wave affects the human brain, the prevailing notion is that alpha, delta, theta, and gamma waves are the most important to learning due to their involvement in areas of the brain traditionally associated with learning [3].
Alpha waves regulate states of consciousness and attention, with sleeping and wakefulness yielding distinct readings [3]. Attention is characterized by a spike in gamma waves triggered by excitatory and inhibitory neurons. These oscillations are also responsible for instigating seeing, hearing, and other sensory responses. Delta and slow waves are linked most closely to sleep; however, groups of neurons that repeatedly signal to one another during sleep have also been found to be associated with memory formation. Additionally, delta waves have been associated with the speed at which rats and other animals learn new tasks. Theta waves are emitted by neurons responsible for encoding memories, prompting the sensory areas of the brain to process and learn information about the environment. Much like how an ocean tide has varying oscillation frequencies made from water, these brain waves are all electrical signals emitted by neurons [1][3].
Furthermore, scientists speculate that each of these waves is produced by or affects specific parts of the brain [1]. Language learning and creativity is associated with the frontal and temporal lobes, particularly with Broca’s region and alpha waves. Math is thought to be correlated with the left frontal lobe and thus is related to beta waves. Movement is thought to be connected to the motor cortex, which is potentially responsible for delta waves. General memory formation and recall are associated with the hippocampus, and the two areas needed for basic animal and human function—the hypothalamus and thalamus—are thought to be the main points of origin for gamma and theta waves. Slow waves, slower waves, and below threshold waves are still being researched and have not been associated with any particular area of the brain [1].
Neural oscillations are usually detected and measured by connecting a patient to an electroencephalography or magnetoencephalography machine (EEG and MEG, respectively) [1][3]. The machines provide a seismograph-like readout of these neural oscillations, similar to how a heart monitor measures cardiac activity. Abnormal EEG and MEG readings allow scientists to better pinpoint potential medical issues, such as epilepsy or sleep disorders [1][3].
However, new research has begun looking at using this technology to alter symptomatic wave amplitudes [1][3]. Researchers have also been studying how to enhance or disrupt learning through changing neural oscillations. They call this new stimulation “wave stimulation” or “wave therapy.”
Dreams
Tracking the evolution of learning through the investigation of neural oscillations began with a simple
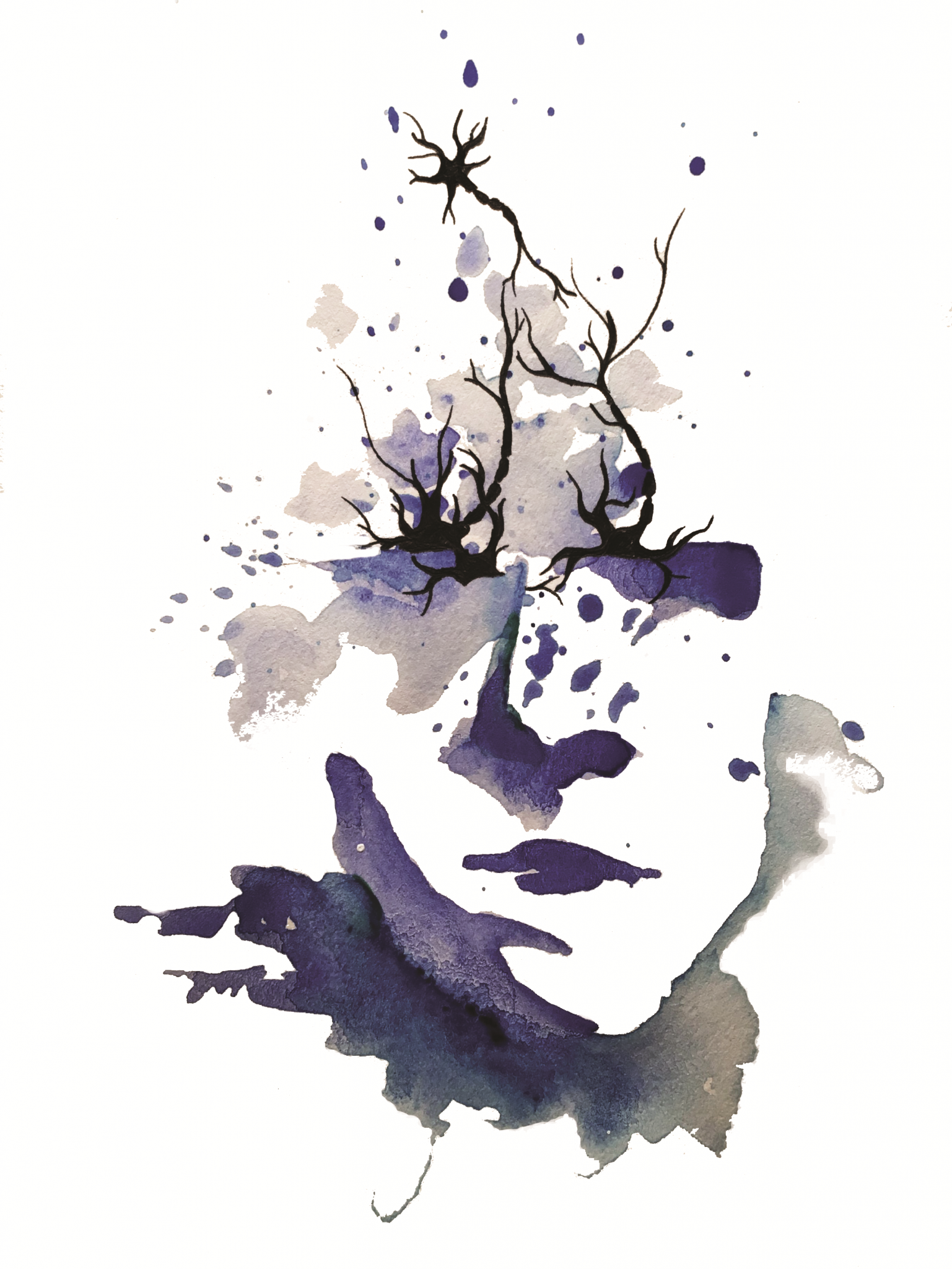
inquiry: How does sleep affect the learning process? In 1926, Dr. Hans Berger of the University of Jena tested human patients with the newly developed EEG and found evidence of distinct electrical signals that could be tracked using the device [4]. He would call these alpha waves. Through sleep deprivation tests done on patients at the university hospital, Berger found that his alpha waves were observed more often when patients had a proper night’s sleep than when they were kept up, noting that their memory seemed impaired in the latter case [4]. This circumstantial finding was considered to be the spark that led Berger to ask how prevalent alpha waves were in learning.
As EEG technology was fine-tuned and the MEG machine made its debut in 1968, researchers at Wright University found that alpha, beta, gamma, theta, and delta waves, although previously detected individually, were observed together for the first time [4][5]. Scientists confirmed previous associations between sleep and learning, finding that subjects’ learning capacities were consistently worse with less sleep than when they were well-rested. From this study, researchers determined that there was at the very least a correlation between types of neural oscillations and learning [4][5].
Learning
Scientists were unsure of how exactly different oscillations interacted in their larger, complex broadband waveform [6]. Part of the community was convinced that certain oscillations were always going to stimulate or downplay other oscillations regardless of which neurons were firing, while others were convinced that the relationship between oscillations was more nuanced and depended on which areas of the brain were firing [6].
Dr. Lawrence Ward of the University of British Columbia was one of the scientists who thought that the proposed MRI and neural oscillation ideas could be merged [6]. He noticed that previous studies had only drawn data from EEG and MEG graphs, which did not account for the continuous processing of information or the oscillations observed during learning, a distinct process of attention, memory encoding, and recall [6].
Ward noted that attention was still linked to alpha waves and also preceded by an increase of alpha wave amplitude. Using EEG and MEG readouts, he saw that alpha wave activity in certain parts of the brain, namely the hypothalamus, was always associated with decreased activity of theta-gamma waves. He also found, however, that the steps of memory encoding and recall had fluctuating trends, with the hippocampus producing theta-gamma and alpha amplitudes in changing amounts. Ward theorized certain waves were inhibiting parts of the brain producing other kinds of waves, such as alpha waves inhibiting theta-gamma wave production. Ward’s research showed that Berger and the researchers at Wright University had both observed the same phenomenon, and Ward had found a unifying model that was in line with both camps’ ideas [6].
What Ward had discovered was a key idea: Brain waves synchronize with one another during various stages of learning, with the amplitude and frequency of waves noticeably changing throughout the process [6]. He hypothesized that learning was a skill that would lead to greater variations in amplitude if trained like any other skill. Ward argued this would help an individual be more attentive and encode and recall faster. Through this argument, he recognized that neural plasticity correlated with clustered patterns of oscillations, leading researchers to ask if waves could be manipulated [6
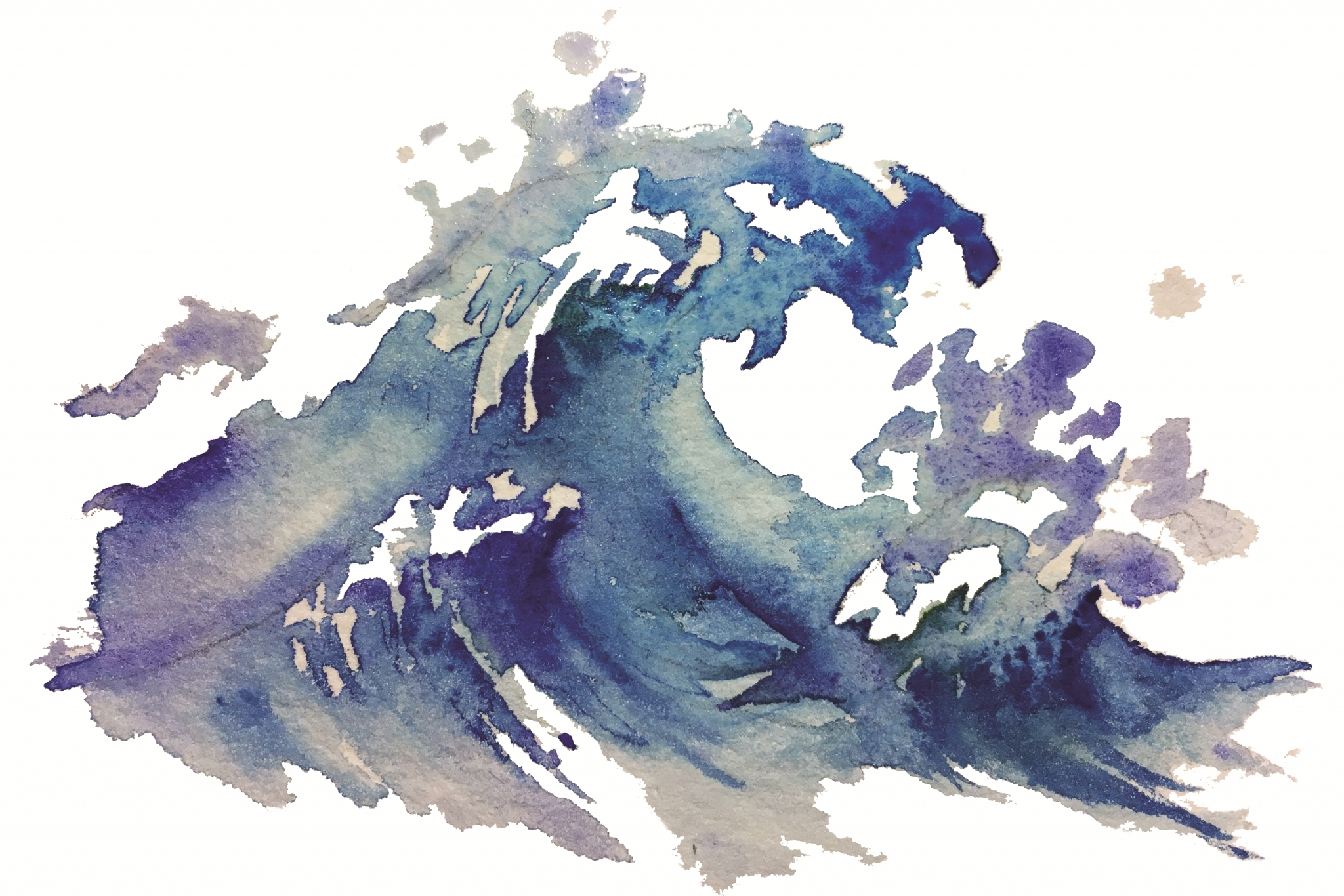
Memory
At the University of Quebec, Dr. Igor Timofeev was pursuing a different lead when he stumbled upon a curious find: Spontaneous slow waves prompted the brain to discard old memories while building new connections with neurons [7].
Timofeev noticed that his review matched the definition of neural plasticity— neuronal connections were continually being removed and rewired to accommodate new information [7]. However, he wanted to figure out whether or not neural oscillations reflected collections of synaptic plasticity and to see how effectively slow waves could change neuronal structure [7].
Timofeev found that animals that received artificial implants to decrease the amplitudes of slow waves were observed to have an increase in gamma and beta waves, suggesting that greater amplitudes of gamma and beta waves would affect targeted neurons more intensely [7]. He expanded on his hypothesis by tracking the differences in human subjects’ theta wave amplitudes while they were awake and asleep. Each patient Timofeev recruited was given a series of cognitive ability tests. He then looked at their EEG readings taken during the tests, particularly their theta wave amplitudes, and found that patients with higher natural theta waves tended to perform better [7].
To see if waves could pair with a cognitive function, scientists at the University of Tubingen began testing whether or not artificial delta wave therapy could increase the learning capacity of individuals’ motor control [8]. More specifically, the researchers believed that the delta stimulation would mimic Ward’s memory consolidation hypothesis.
Scientists took young, healthy adult volunteers and taught participants to use a machine that would track their delta wave activity while viewing an image related to movement [8]. Choosing delta waves was a deliberate choice, as previous studies showed an increase in delta waves during movement-related activities, like playing soccer or the piano, so researchers knew their machine and observations would sync up. The setup was elegant: If a subject’s delta amplitude was detected in sufficient amounts, the machine’s computer would open up a mechanical hand to reflect a physical motion. To mitigate the natural variance of delta waves that would cloud up the data, scientists split the volunteers into groups that would receive minimal delta stimulation, more delta stimulation, or no stimulation in order to fairly assess the potential difference between each of the groups [8].
By the end of the five-day experiment, individuals who received the stronger delta-wave stimulation were consistently able to open the mechanical hand better than those with minimal or no delta wave stimulation [8]. Moreover, during a thirty-day follow-up, individuals who had experienced the stronger stimulation were still doing better at the task, even without practicing. This proved promising, as it suggested that delta waves had an effect on memory consolidation and recall [8]. The study also demonstrated that stimulation might have further applications wherein oscillations could help with other cognitive functions.
Math and Monkeys
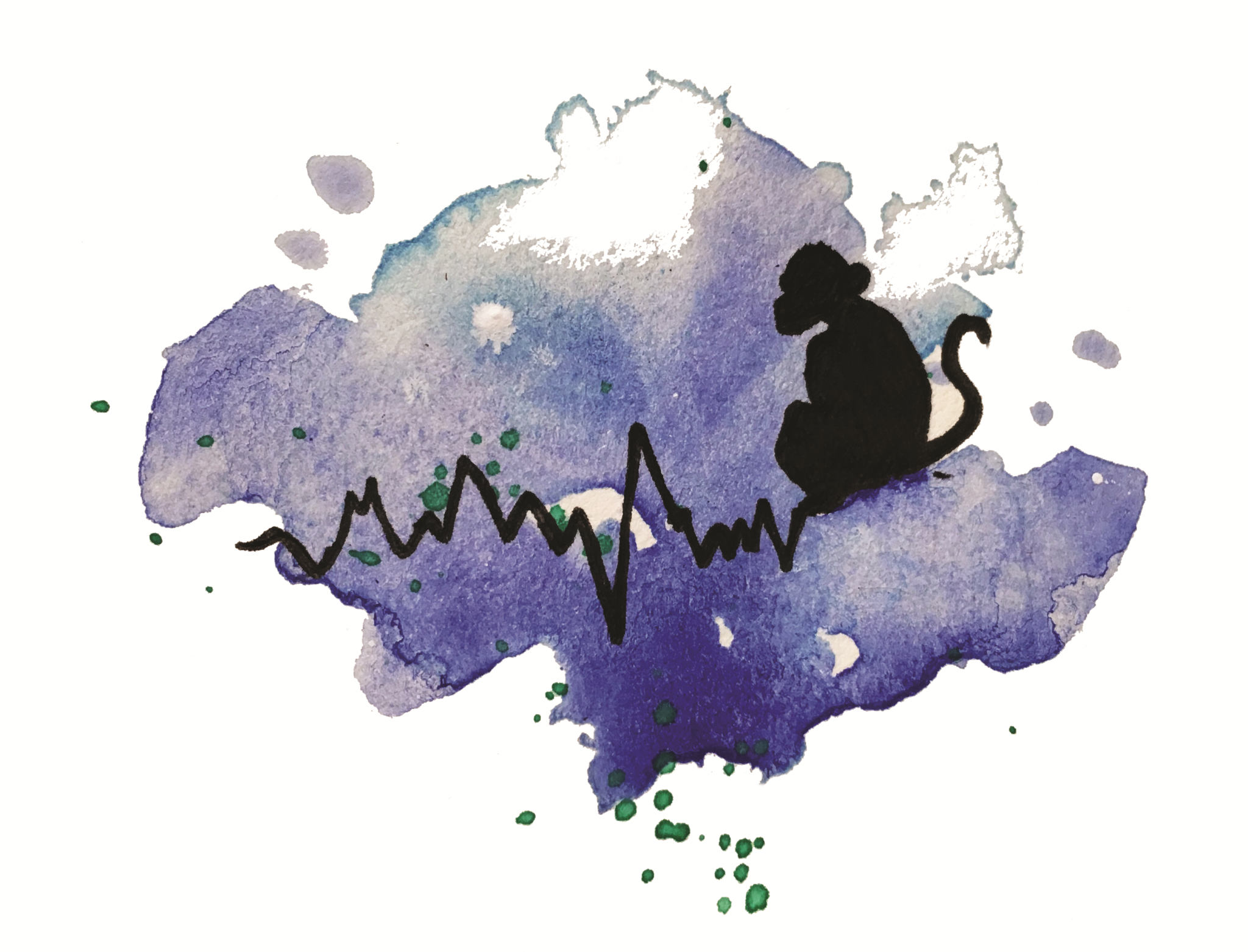
At the onset of these discoveries, Dr. Earl Miller of MIT was curious about the individual functions of each kind of wave [9]. Working from the principles other scientists had established, he started teaching rhesus monkeys to count mundane objects while tracking how well the waves lined up in order to see how closely the monkeys’ brain waves would match their cognitive abilities [9].
To test this, Miller had the monkeys learn to track the number of items they saw on-screen by tapping a touch pad and then showed them new images with a similar placement of objects to ensure they were actually counting [9]. Four stages were used to see how the monkeys’ brain waves would change throughout the learning process.
The test was repeated three separate times, with each trial tracking spikes in activity to identify which frequency would be associated with learning spatial reasoning and basic math [9]. Miller’s other experiments indicated that there was an association between physical movement and memory, suggesting there would be some change in gamma waves. Indeed, Miller found evidence of communication between certain neurons during the math tests that correlated with increased gamma wave activity. Subsequently, he theorized that these neurons were sending more waves to build motor connections so that the monkey’s fingers moved to the correct answer, which would correlate with the emission of fewer beta waves. Additionally, Miller thought that a monkey taking these tests would display greater amounts of gamma waves due to neural plasticity than would a monkey without prior testing experience, so taking baseline tests could help spot monkeys who might have higher scores during the initial rounds [9].
However, what Miller did not expect was that only the gamma waves would modulate greatly regardless of whether the monkeys were counting; the gamma and beta waves fluctuated at inversely proportional amplitudes and in alternating times [9]. Neurons firing for movement meant that monkeys were self-correcting mistakes they made while tapping and learning to coordinate movement. The math trend network and selection neurons in turn were showing increased levels of gamma waves and decreased beta signatures each time a monkey performed the same task, as was expected [9].
Meanwhile, neurons were producing greater amounts of gamma waves, emitting them at higher amplitudes since the brain was building greater neural connections due to the continual presentation of new information during the test. To Miller, this suggested that there was a spacing between neural oscillations that could help isolate each type with a specific cognitive task. Through his experiment, Miller realized that a task could be continually performed in order to strengthen an individual’s neuronal connections related to the skill and thus increase or decrease the waves. He figured that by tracking an individual’s skill learning over time and matching it with EEG or MEG scans of neural oscillations, he could pinpoint the specific waves responsible for strengthening that task [9].
As he analyzed the data, Miller found that the monkeys were all emitting lower levels of beta waves and greater amounts of gamma waves with each trial, suggesting that they were learning to move their hands in response to the images being shown with less delay and greater focus. With this study, Miller found that individual waves could be isolated by performing certain tests, an idea that would be further teased apart with the inclusion of various testing protocols [9].
Waves and Creativity
Dr. Flavio Frohlich of University of North Carolina at Chapel Hill was struck by the idea of testing waves correlated with creativity [10]. Thumbing through prior research about higher alpha wave readings in creatives than the average person, he decided to explore whether or not there was a connection between alpha waves and creativity. From the literature, Frohlich knew there was evidence of an alpha wave-creativity association. To best test this idea, Frohlich began assessing how well participants could construct pictures and complete pictures with given parts, among other measures found in the Torrance Test of Creative Thinking (TTCT) [10].
The participants were initially tasked with completing one of the 30-minute TTCT tests while either a sham, weak-amplitude, or low frequency alpha wave or a strong amplitude and high frequency alpha wave therapy was administered [10]. Frohlich’s study analyzed how well subjects performed compared to their own prior results so he could better understand how each type of oscillation stimulation might boost or harm a participant’s baseline creativity. Researchers found that individuals who received the larger amplitudes of alpha stimulation did noticeably better on the TTCT tests compared to their initial tests. Moreover, their brain scans indicated higher alpha wave activity after the stimulation sessions [10]. This was an important discovery that suggested there was not only a direct link between creativity and alpha wave therapy, but also possible benefits of stimulation in novel skills [10].
Researchers are unsure of how the alpha waves emit from the parts of the brain responsible for creativity since these parts of the brain have not been found yet [10]. Alongside these prior issues, the stimulation was not replicated with the same subjects more than once, making it difficult to determine whether this effect was long-term or merely a fluke [10].
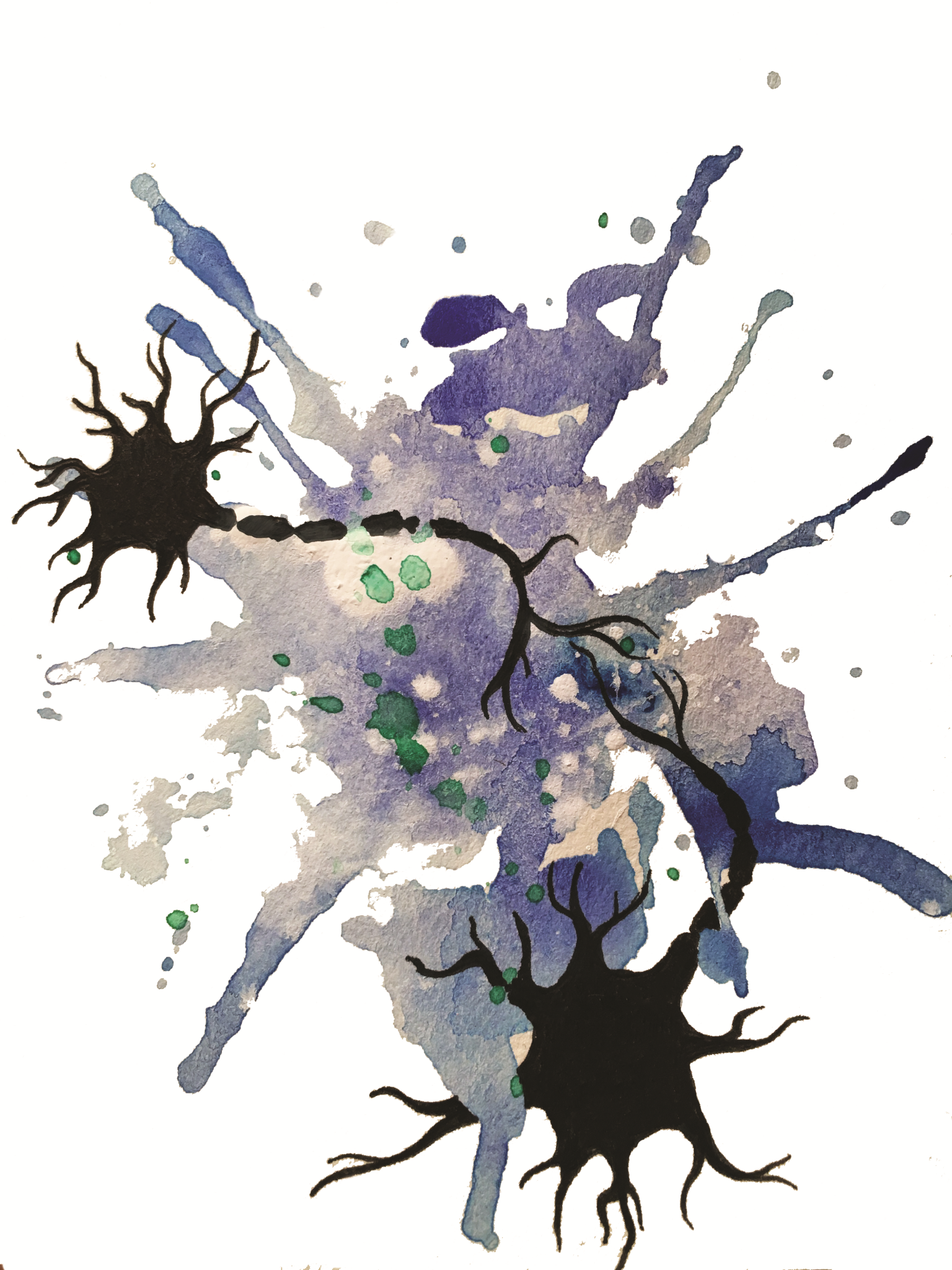
A Future Unknown
The testing and improvement of alpha wave stimulation necessitates further exploration and understanding of the neural oscillations associated with learning [10]. Scientists continue to create ethical guidelines for the burgeoning field, with wave therapy serving as a model for how future neural engineering will be tested, developed, and distributed [11].
Scientists must refine the imaging techniques used to better understand the different types of waves and effects they have in wave therapy [11]. Current methods may be considered safe, but they fail to elucidate the actual regions of the brain being stimulated by the wave machines [10]. Rather, if scientists want to find a clearer answer, they must look toward either invasive methods, such as implanting sensors, or pursue new ways to look into the brain without harming an individual’s health [11].
Additionally, researchers have specified that the technology must serve a beneficial role in helping patients [11]. Traditionally, treatments using electrical impulses involve implanting electrodes into a patient’s brain. However, this has often led to needless complications that would harm the patient, such as short circuiting or surgical errors. Due to these risks, researchers have been pursuing safer alternatives, like wave stimulation, to move away from technologies that might endanger patients [11].
When devising new experiments and techniques to make wave stimulation safer, scientists want to avoid the long-term toxicity, size, and misuse of data collection that past iterations of electrodes and other devices have encountered [11]. This has become particularly relevant for alterable technologies such as brain computer interfaces, which, like wave stimulations, are altering the baseline capabilities of the patient. Scientists cite the previous attempts to create electrodes that help stroke patients as a standard that should be met when developing and testing the side effects and benefits of oscillation stimulation. Although they hypothesize the benefits stimulation could have on learning, scientists are wary about the long-term changes that might be lurking in the future. Deciding to pursue alternative methods or work to improve such technologies is an ongoing logistical challenge for researchers in this field [11].
However, the greatest concern for ethicists is the privilege of access this technology will set the precedent for [11]. Historically, forcibly pushing technology on underserved or disadvantaged groups was the strategy employed by researchers to test and treat persons with ailments.
Scientists must be fully cognizant of how they select people who would need this wave stimulation most while considering both their safety and their consent to such testing [11]. If the stimulation were to become a tool meant to treat people with learning disabilities, the scientific community must be aware of the patient’s or intended group’s desire. Without the social and legal considerations, the entire endeavor could be called into question and regarded as a possible technological and systemic failure [11].
However, scientists have developed new approaches to mitigate these social issues by actively engaging the communities they aim to serve [11]. In the case of learning stimulation, this method has entailed recruiting willing participants who are told about the procedure and potential risks. Through this dialogue, scientists are better able to understand the user experience while also identifying user-specific issues with the oscillation stimulation.
Wave stimulation may produce exciting studies, but the few that exist have not been replicated to determine their legitimacy and their results’ causational significance. Aside from possible applications in learning creativity that may prove promising, scientists have also opened a new Pandora’s box that requires careful ethical attention. Despite all the quandaries, however, these studies may serve as a pioneering foray into new avenues for neural engineering that will enhance and challenge the way humans live and learn.
- Moran, L. V., & Hong, L. E. (2011). High vs Low Frequency Neural Oscillations in Schizophrenia. Schizophrenia Bulletin, 37 (4), 659-663. doi:10.1093/schbul/sbr056
- Spruston, N. (2008). Pyramidal neurons: Dendritic structure and synaptic integration. Nature Reviews Neuroscience, 9 (3), 206-221. doi:10.1038/nrn2286
- Zhang, X., Lei, X., Wu, T., & Jiang, T. (2013). A review of EEG and MEG for brainnetome research. Cognitive Neurodynamics, 8 (2), 87-98. doi:10.1007/s11571-013-9274-9
- Shepard, J. W., Buysse, D. J., Chesson, A. L., Dement, W. C., Goldberg, R., Guilleminault, C., … White, D. P. (2005). History of the Development of Sleep Medicine in the United States. Journal of Clinical Sleep Medicine : JCSM : Official Publication of the American Academy of Sleep Medicine , 1 (1), 61–82.
- Bonnet, M. H. (1987). Sleep Restoration as a Function of Periodic Awakening, Movement, or Electroencephalographic Change. Sleep, 10 (4), 364-373. doi:10.1093/sleep/10.4.364
- Ward, L. M. (2003). Synchronous neural oscillations and cognitive processes. Trends in Cognitive Sciences,7 (12), 553-559. doi:10.1016/j.tics.2003.10.012
- Timofeev, I. (2011). Neuronal plasticity and thalamocortical sleep and waking oscillations. Progressive Brain Research, 193 , 121-144. doi:10.1016/B978-0-444-53839-0.00009-0
- Soekadar, S. R., Witkowski, M., Birbaumer, N., & Cohen, L. G. (2014). Enhancing Hebbian Learning to Control Brain Oscillatory Activity. Cerebral Cortex, 25 (9), 2409-2415. doi:10.1093/cercor/bhu043
- Lundqvist, M., Herman, P., Warden, M. R., Brincat, S. L., & Miller, E. K. (2018). Gamma and beta bursts during working memory readout suggest roles in its volitional control. Nature Communications, 9(1). doi:10.1038/s41467-017-02791-8
- Lustenberger, C., Boyle, M. R., Foulser, A. A., Mellin, J. M., & Fröhlich, F. (2015). Functional role of frontal alpha oscillations in creativity. Cortex, 67 , 74-82. doi:10.1016/j.cortex.2015.03.012
- Moritz, C. T., Ruther, P., Goering, S., Stett, A., Ball, T., Burgard, W., . . . Rao, R. P. (2016). New Perspectives on Neuroengineering and Neurotechnologies: NSF-DFG Workshop Report. IEEE Transactions on Biomedical Engineering, 63 (7), 1354-1367. doi:10.1109/tbme.2016.2543662