Vision is a sensory system that we rely on heavily, and yet many people face visual impairments. The National Eye Institute recently reported that there were over 4 million people with visual impairments in the United States, mostly due to disease (NEI). Many diseases affect the retina that can lead to permanent blindness, and these typically involve the loss of neural cells. Although most people think about vision deterioration as a normal part of the aging process, there are non-mammalian vertebrates that can regenerate damaged cells in their retina. In studying this pathway, there is the possibility of treating or even curing these diseases.
Though it is in the eye, the retina is a part of the central nervous system (CNS). It is composed of neurons that receive visual input and transmit that information to the visual areas of the brain. Light passes through the outer layers of the retina to reach the photoreceptors, and these cells, divided into rods and cones, translate this light into an electrical signal with light-sensitive proteins. Other cells in the retina then take this input, combine it to start processing the visual information, and transmit it back to the visual cortex via the optic nerve. The other important cell type in the retina are Muller glia—they work to support the structure and function of the retina and are currently considered to be mainly helper cells. It is this type of cell that holds the potential to treat retinal diseases.
Many non-mammalian vertebrates have the ability to regenerate neuronal cells in response to damage in the retina [1]. Zebrafish have been studied due to the fact that their entire genome has been sequenced and have other regenerative capabilities as well [2]. Human and Zebrafish retinas both have the same cell types and functional organization, as well as some similar protein expression patterns [1]. However, Muller glia in the Zebrafish retina have the ability to go backwards in their developmental cycle to a more stem-cell like state, called progenitors, and these divide to produce new neurons [3]. Zebrafish trigger this pathway in response to retinal damage, but mammals do not have this ability to make new cells in the retina to replace those lost from injury or disease.
Zebrafish retinal development and regeneration has the potential to change our ability to treat humans with degenerative eye diseases. One transcription factor that is specifically expressed in response to damage in Zebrafish cells is Ascl1 [4]. Previous work has shown that Müller glia in mice can also be made to produce some new neurons after retinal damage, with experimental Ascl1 gene overexpression using a virus or genetic modification [5]. This illustrates promising evidence that some of the elements involved in fish retinal regeneration can be applied to mammals as well. With a greater understanding and application of this pathway in Zebrafish, could our eyes heal themselves in the same way?
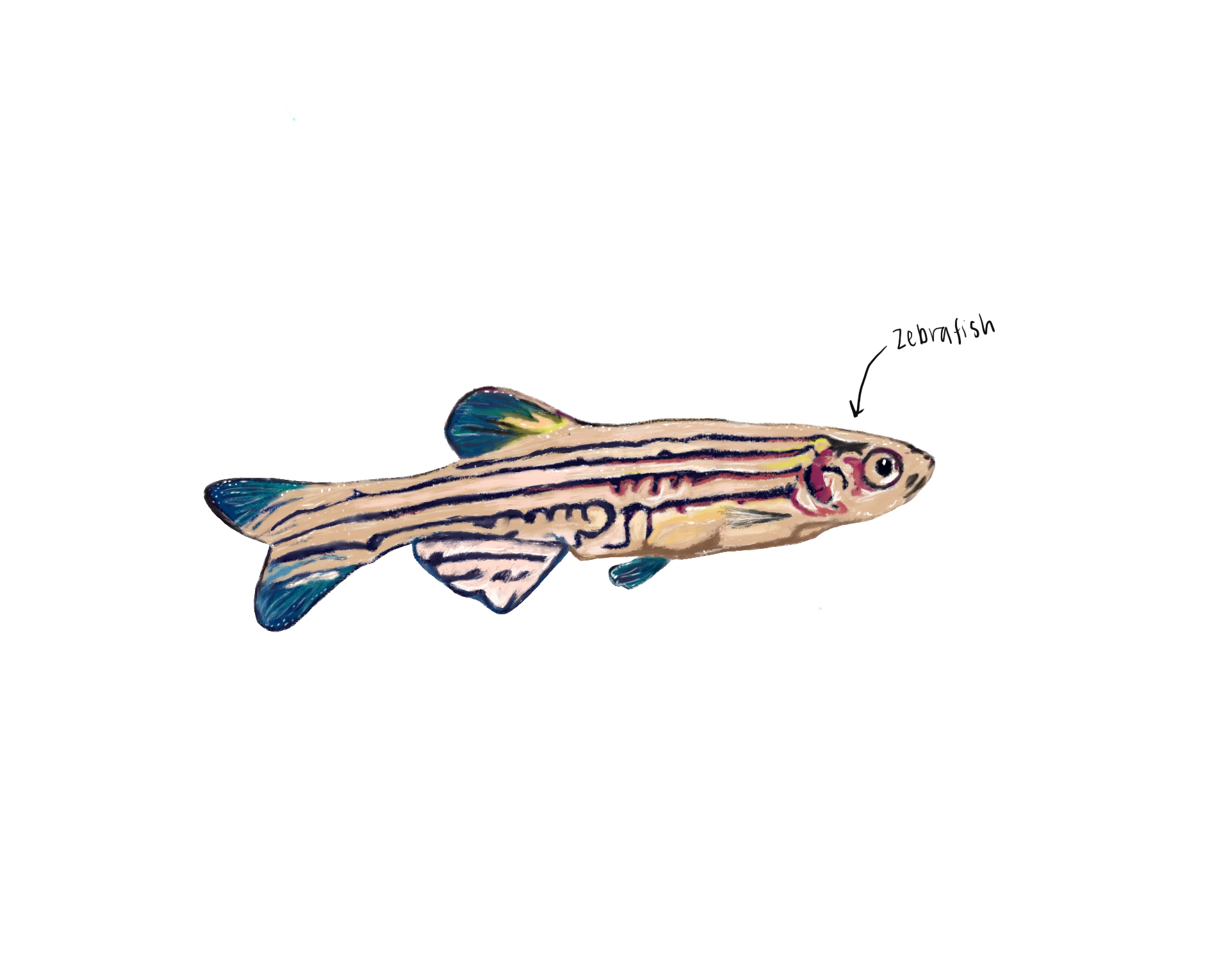
Genetic Pathway
Genetic editing techniques are seen as a way to treat diseases when drugs cannot be found. While “correcting” the mutation involved in a disease pathway is a potential cure to some diseases, if they are linked to a specific mapped gene, another way to approach the problem would be to push human retinal cells into the same regeneration pathway that occurs in Zebrafish. In this pathway, Muller glia respond to damage by upregulating some genes, which drive the cell towards a more stem-like state [3]. These progenitors then begin to divide into neural cells due to different factors, restoring the lost or damaged cell types in the retina. There are many genes found to be involved in the pathway so far, such as the Map Kinase and Notch signaling pathways, and Ascl1 [3].
Ascl1 upregulation, or a forced increase in expression, has been shown to be necessary in Zebrafish for producing replacement neural cells, and this transcription factor, which manages other gene expression, is a very central part of the pathway [3]. In characterizing the different genes involved, one study stopped Ascl1 production after retinal injury in Zebrafish, which prevented the production of any progenitors or neural cells [4]. This, along with the fact that mice do not normally express Ascl1 after damage even though they possess the gene, suggests that Ascl1 is required to drive Muller glia into the regeneration pathway. Overexpression of Ascl1 in mice with damaged retinas can also drive the production of new neural cells [5].
Although Ascl1 is necessary in mice to produce new neurons, manipulating other genes in the pathway would be necessary to robustly replace damaged cells. In the regeneration pathway, the immediate response to damage is an upregulation in several growth factors [3]. Changes in signaling cascade pathways activated due to the damage have also been shown to be involved in regulating the glial response, and these may occur in response to damage or as a consequence of the upregulated growth factors. This is a complex pathway, as over 1500 genes have been identified with changed expression in the progenitors versus Muller glia [3].
Primate Research on Color Blindness
Primates are incredibly useful in further testing potential clinical therapies, as they are similar to humans and can be used to more accurately predict the spread of viral vectors in human eyes. Unlike other mammals, primates have a macula, the part of the retina responsible for most of our color and high-resolution vision, so they are especially important in testing treatments for diseases such as age-related macular degeneration [6]. However, to fully understand specific retinal diseases and treatments, a genetic model of the disease is necessary to test treatments. There are a few primate models of human retinal diseases, but similar symptoms can also be experimentally produced to test the effects of viral treatments [6].
AAVs have successfully been used to deliver genes to the primate retina to cure colorblindness. One example of a genetic model very similar to humans occurs are squirrel monkeys, as males of this species are red-green colorblind, because they lack the L-opsin gene. Although colorblindness is not a degenerative disease, it does affect 1 in 12 men, and can prevent people from working in certain vocations (NEI). In one study, gene therapy to replace the L-opsin gene was shown to give the squirrel monkeys the ability to differentiate red and green [7]. Monkeys were initially trained to perform a modified version of the Cambridge Color test, a computer-based test used to determine color blindness in humans. These monkeys were shown to be unable to distinguish red and green as a baseline. After an AAV L-opsin gene was injected, however, they were able to accurately pick red and green, One interesting aspect of this study, however, was that it actually took 20 weeks after the injection for trichromatic vision to appear in testing [7]. This timeline suggests that in other studies, and for genetic therapies, the amount of time needed for cells to take in DNA and begin expressing it, or perhaps the other changes needed cellularly, may occur at varying amounts of time.
Gene Therapy Clinical Trials
There are many diseases that affect the retina, these include: Age-Related Macular Degeneration, Cataracts, Glaucoma, and Diabetic Retinopathy (NEI). Unlike degenerative diseases of the brain, the retina is theoretically easier to restore due to its size and location. This makes the retina a good target for clinical intervention, as it is more isolated and so can receive foreign material without having as much of an immune response [8]. Some specific diseases have been genetically mapped out more clearly, and are closer to becoming curable.
Retinal regeneration research has focused on investigating how Zebrafish are able to produce more cells, and to replicate this ability in mice and other animals, with the goal of eventually being able to use this knowledge to develop therapies in humans [3]. Current clinical trials are focused on using stem cell therapy to treat retinal degenerative diseases, but there is also research focused on producing neurons from mammalian Muller Glia in a pathway similar to the one that Zebrafish use [9]. This therapy uses viruses to transfer DNA to diseased or other cells.
Viruses are used for gene therapy because they can effectively infect cells with specific DNA. The term viral vector is used to describe viruses which are stripped of their replication ability so that they don’t spread but can still infect the target cells and are edited to deliver specific genes. With this DNA addition, the machinery to read DNA in the cell will treat the new DNA as its own and produce the protein it codes for. In cases where a disease is caused by a faulty gene, this can be used to put in a working copy. Experimental overexpression can be achieved by infecting cells with a gene they may already have, so that more of the protein is produced. Although there are a couple types of viral vectors, an important one that has been used in clinical trials are adeno-associated viruses or AAVs.
For example, the first retinal degeneration disease to have a gene replacement therapy treatment was Leber Congenital Amaurosis (LCA) [10]. There are 26 genes that have been found to be associated with LCA, and for LCA subtype 2, a genetic therapy using the aforementioned AAVs was approved and went into clinical trials in 2008. Luxterna ® was the first FDA-approved gene therapy for a genetic disease, specifically for patients with a mutation in a gene called RPE65 which leads to the LCA subtype 2 [10]. It is, however, a very expensive treatment (about $450,000 per eye) with limited efficacy, which brings into question the ethics of this treatment. We owe it to the visually impaired to make a more inexpensive treatment, as we live in a very vision-oriented world.
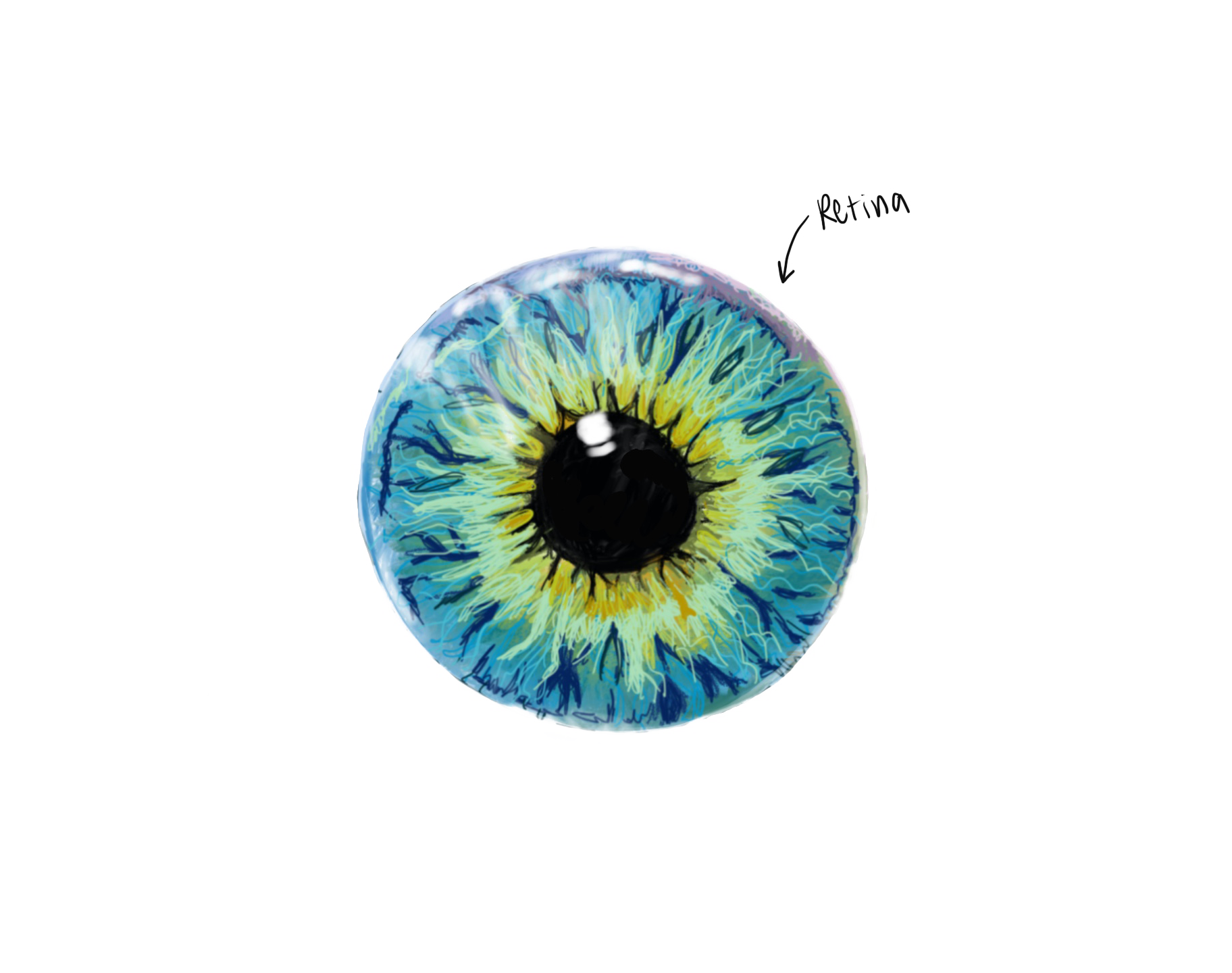
Conclusion
It could be possible, in humans, to manipulate whatever proteins are sufficient to direct Muller glia into a neuronal cell producing pathway with some overexpression viruses. FDA trials have shown that although not fully effective, viral vectors are a safe vehicle for genetic overexpression, and with the right targets would be more effective. While neural cells have been produced from Muller glia in mice, the question of how to produce all of the specific cells in the retina remains.
Diseases with one specific cell type damaged, such as in LCA with affected pigmented retinal epithelium cells, may be more difficult to produce by following the zebrafish regeneration pathway, but with a better understanding of the factors involved as well as disease genetics, the needed cells may be produced. There are ongoing clinical trials for diseases such as Retinitis Pigmentosa using other AAVs, as well as for transplanting developed cells from human stem cells for Stargardt Maculopathy [8]. Understanding the pathway in mammalian models such as mice and primates potentially gives us the ability to then produce needed cell types in humans with virus mediated delivery of needed genes or factors. The fact that retinal injections of genetic therapies are possible without major side effects, means that with the right target and vector, there is the potential to restore eyesight.
In moving to human trials, one main focus will be whether newly made neurons integrate with the visual system. In order for retinal regeneration to be useful, patients with retinal diseases should also be able to process the visual information that their new retinal cells may receive. Patients with visual impairments may have lost some of that wiring from lack of use. A more studied example of this occurs in the auditory area of the brain, as cochlear implants provide new input that needs to be integrated into the existing processing of auditory information. These implants work much better at younger ages, as the auditory cortex is able to adapt to the new signals coming in from the cochlea [11]. Similarly, with genetic editing techniques for restoring vision, the rest of the pathway needed to understand an image is taken into account to truly restore sight. Through the different approaches scientists are taking, studying Zebrafish, mice, primates, and finally testing these in clinical trials, it is possible that there may soon be cures for many retinal diseases.
References
- Avanesov, A., & Malicki, J. (2010). Analysis of the retina in the zebrafish model. Methods in cell biology, 100, 153–204. https://doi.org/10.1016/B978-0-12-384892-5.00006-2
- Jopling, C., Suñé, G., Faucherre, A., Fabregat, C., & Izpisua Belmonte, J. (2012). Hypoxia induces myocardial regeneration in zebrafish. Circulation, 126(25), 3017-3027.
- Goldman, D. Müller glial cell reprogramming and retina regeneration. Nat Rev Neurosci 15, 431–442 (2014). https://doi.org/10.1038/nrn3723
- Fausett, B. V., Gumerson, J. D., & Goldman, D. (2008). The proneural basic helix-loop-helix gene ascl1a is required for retina regeneration. The Journal of neuroscience : the official journal of the Society for Neuroscience, 28(5), 1109–1117. https://doi.org/10.1523/JNEUROSCI.4853-07.2008
- Pollak, Julia et al "ASCL1 reprograms mouse Müller glia into neurogenic retinal progenitors." Development 140.12 (2013): 2619-2631. Web. 05 April. 2020.
- Picaud, S., Dalkara, D., Marazova, K., Goureau, O., Roska, B., & Sahel, J. (2019). The primate model for understanding and restoring vision. Proceedings of the National Academy of Sciences of the United States of America, 116(52), 26280-26287.
- Mancuso, K., Hauswirth, W. W., Li, Q., Connor, T. B., Kuchenbecker, J. A., Mauck, M. C., . . . Neitz, M. (2009). Gene therapy for red–green colour blindness in adult primates. Nature, 461(7265), 784-787. doi:10.1038/nature08401
- Ziccardi, L., Cordeddu, V., Gaddini, L., Matteucci, A., Parravano, M., Malchiodi-Albedi, F., & Varano, M. (2019). Gene Therapy in Retinal Dystrophies. International journal of molecular sciences, 20(22), 5722. https://doi.org/10.3390/ijms20225722
- Ramsden, C. M., Powner, M. B., Carr, A. F., Smart, M. J., Da Cruz, L., & Coffey, P. J. (2013). Stem cells in retinal regeneration: Past, present and future. Development, 140(12), 2576-2585. doi:10.1242/dev.092270
- Testa, F., Maguire, A., Rossi, S., Pierce, E., Melillo, P., Marshall, K., . . . Simonelli, F. (2013). Three-Year Follow-up after Unilateral Subretinal Delivery of Adeno-Associated Virus in Patients with Leber Congenital Amaurosis Type 2. Ophthalmology, 120(6), 1283-1291.
- Sharma, Anu; Dorman, Michael F.; Spahr, Anthony J. A Sensitive Period for the Development of the Central Auditory System in Children with Cochlear Implants: Implications for Age of Implantation, Ear and Hearing: December 2002 - Volume 23 - Issue 6 - p 532-539
- Ueki, Yumi et al "Transgenic expression of the proneural transcription factor Ascl1 in Müller glia stimulates retinal regeneration in young mice." Proceedings of the National Academy of Sciences 112.44 (2015): 13717-13722. Web. 05 April. 2020.
- Wilken, M., & Reh, T. (2016). Retinal regeneration in birds and mice. Current Opinion in Genetics & Development, 40, 57-64.
- Tsuruma, K., Saito, Y., Okuyoshi, H., Yamaguchi, A., Shimazawa, M., Goldman, D., & Hara, H. (2018). Granulin 1 Promotes Retinal Regeneration in Zebrafish. Investigative Ophthalmology & Visual Science, 59(15), 6057-6066.
- Kaplan, H., & Fernandez de Castro, J. (2012). Retinal regeneration and stem cell therapy in retinitis pigmentosa. Taiwan Journal of Ophthalmology, 2(2), 41-44.
- Conner, C., Ackerman, K., Lahne, M., Hobgood, J., & Hyde, D. (2014). Repressing notch signaling and expressing TNFα are sufficient to mimic retinal regeneration by inducing Müller glial proliferation to generate committed progenitor cells. The Journal of Neuroscience : The Official Journal of the Society for Neuroscience, 34(43), 14403-14419.
- Nakamura, K., & Chiba, C. (2006). Roles of notch signaling in retinal regeneration of adult newt. Zoological Science, 23(12), 1179.
- DuVal, M., & Allison, W. (2017). Impacts of the retinal environment and photoreceptor type on functional regeneration. Neural Regeneration Research, 12(3), 376-379.
- Mcginn, T., Leoni, D., Partington, N., & Stenkamp, D. (2016). Restoration of the dendritic fields and cone synapses of bipolar cells during retinal regeneration in the zebrafish. Investigative Ophthalmology & Visual Science, 57(12), Investigative Ophthalmology & Visual Science, 2016 Sep, Vol.57(12).
- Bainbridge, J., Smith, A., Barker, S., Robbie, S., Henderson, R., Balaggan, K., . . . Ali, R. (2008). Effect of Gene Therapy on Visual Function in Leber's Congenital AmaurosisBrief Report:. The New England Journal of Medicine, 358(21), 2231-9.
- "Facts About Color Blindness". NEI. February 2015. Archived from the original on 28 July 2016. Retrieved 29 July 2016.