Introduction
One of the major functions of the nervous system is to control muscles and coordinate their activity to generate movement. Most motions we execute are a product of sensory input followed by motor output, which initiates coordinated muscle contraction. The structures involved in this process are simple at the microscopic level: typically, one motor neuron is connected to and directly stimulates a single muscle fiber at the neuromuscular junction [1] . However, the formation of the neuromuscular junction requires a vast array of signals that precisely direct the formation of each of the billions of neurons in our bodies and guide them to their final destinations [2] . The challenge for the body is to form the correct type of neuron in the proper location and direct axonal growth to the intended target, which in some cases can span over a meter in length. Neurodevelopment resembles a staircase in nature; its stages are the steps that must be climbed in order to reach the top. At the bottom of the staircase is the fertilized egg, from which the development of a motor neuron, such as that of a bicep muscle, can be traced to the top, where the neuromuscular junction becomes a functional structure.
The Formation of the Primitive Neural Tube
As a single fertilized egg develops into an embryo, it will undergo successive cell divisions and morphological changes until it becomes a hollow ball of cells called a blastula. In mammals, the blastula will invaginate, or fold in upon itself, to form the gastrula, which consists of the primitive gut and the three germ layers called the ectoderm (outer layer), mesoderm (middle layer), and endoderm (inner layer). Within the ectoderm, there is a specialized group of cells called neural plate cells that are bordered by epidermis. The formation of neural plate cells is induced by signaling molecules released from the underlying notochord, a rod-like structure spanning the length of what will eventually become the spinal cord. During neurulation, the neural plate folds in upon itself to form the neural tube, the precursor to the brain and spinal cord [3] .
The Formation of the Spinal Motor Neuron
When neurulation is complete, the neural tube contains a hollow center and is covered by a layer of epidermis [1] . The hollow center of the neural tube is surrounded by the ventricular zone, where neural progenitor cells divide and migrate towards the sides of the neural tube into the intermediate layer. Here, they mature into either neurons or glial cells. Their fate is determined by the release of signaling factors – molecules that act as a form of communication – from the dorsal roof plate and the ventral floor plate, as well as by their position within the neural tube [1] .
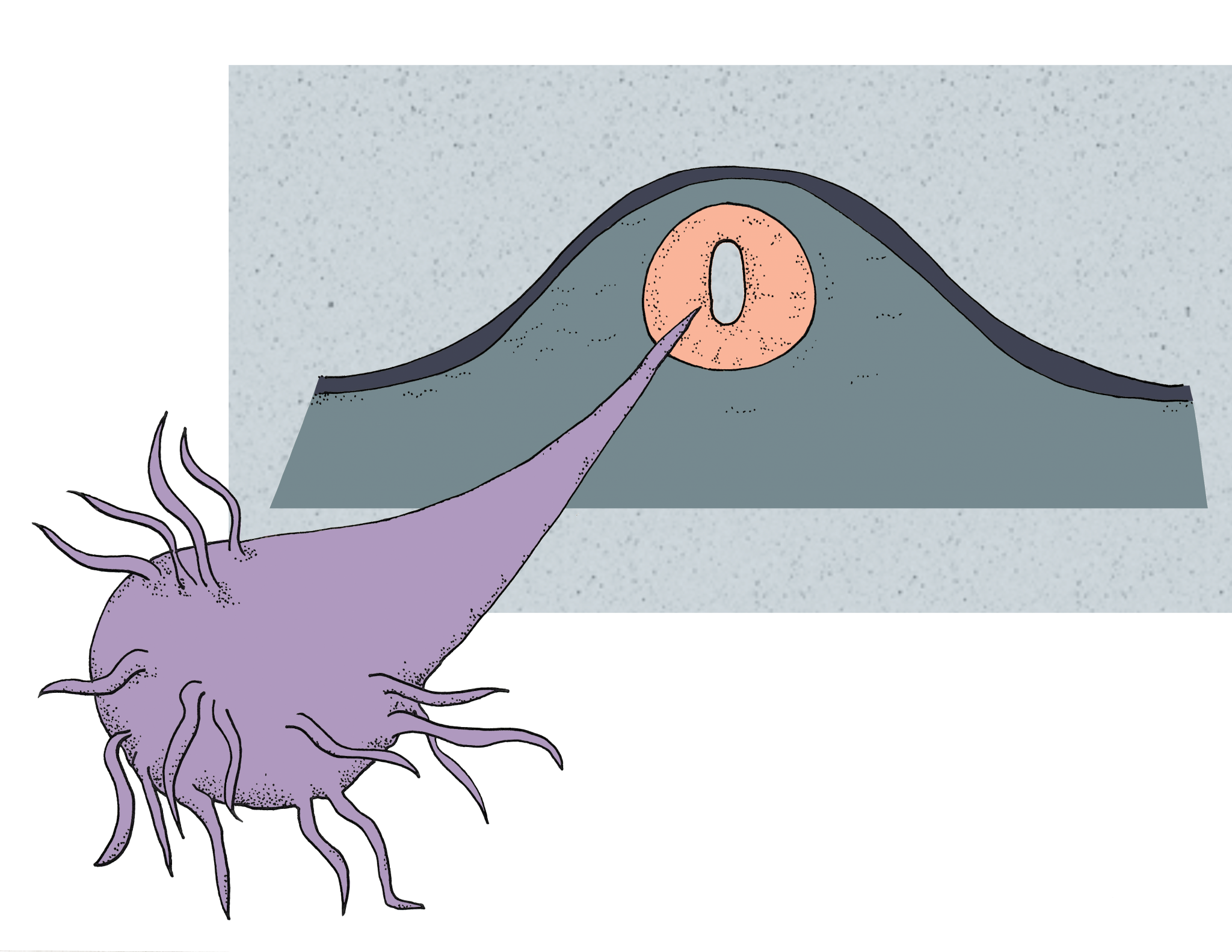
While there are many signaling factors involved, the two most heavily studied factors are the bone morphogenetic protein (BMP) released by the roof plate and sonic hedgehog (SHH) released by the floor plate. Interestingly, the sonic hedgehog gene was named after the video game character, Sonic, because other members of the protein family had names related to actual types of hedgehogs, such as Indian and desert [4] . The release of BMP and SHH from the plates form concentration gradients within the neural tube. A concentration gradient is commonly pictured as a pyramid, where the highest amount of a signaling factor is found at the base, representing the source of the factor. The amount decreases as the height of the pyramid increases. Our future spinal motor neuron is located near the floor plate, meaning it is located near the base of the SHH pyramid and near the top of the BMP pyramid. Along with many other signaling factors, high SHH and low BMP levels induce the production of transcription factors that activate certain genes. Transcription factors are proteins that bind to particular DNA sequences, called enhancers or promoters, during transcription and regulate gene expression based on the sequence they bind to. For example, the high SHH and low BMP levels will cause the progenitor cell for our neuron of interest to synthesize transcription factors that enhance transcription of genes required for the formation of a motor neuron as well as transcription factors that repress genes for sensory neuron development. In contrast, neural progenitors near the roof plate encounter high BMP and low SHH levels, causing them to express proteins for sensory neuron development. This gives rise to the divisions of the spinal cord, where sensory neurons are located dorsally and motor neurons are located ventrally [4] .
The fate of neurons in the neural tube is also determined along the anterior-posterior axis, or top-to-bottom, in addition to the dorsal-ventral axis, or back-to-front. Along the neural tube to the bottom of the primitive brain, there exists a concentration gradient of signaling factors like retinoic acid or fibroblast growth factors [5] . These stimulate the selective expression of Hox genes, which dictate the identity of neurons within the neural tube based on location. Hox genes are very similar to the structure of a worm in that they are segmented. Although Hox genes exist throughout the neural tube, only select combinations of Hox genes are expressed in defined segments. In the case of our future motor neuron, the cell would be located in the brachial segment of the neural tube. The Hox6 gene is primarily expressed in this region, causing the production of transcription factors that activate additional genes related to controlling muscles in the forelimbs and the chest. Due to the expression of Hox6, our motor neuron develops as part of the lateral motor column, from which it will send its axon out to the limbs. Other muscles in the body are innervated by motor neurons in other distinct motor columns [5] .
Innervation of the Forelimb
In order for our motor neuron to reach the arm, it must extend its axon from its cell body, located in the neural tube, to the developing forelimb. As the motor neuron matures from a neural progenitor, changes in the combination of transcription factors initiate axonogenesis, or the formation of an axon [6] . Motor neurons exit the basal plate of the neural tube at various lateral locations based on what set of transcription factors is activated. At this stage of development, the caudal portion of the neural tube is maturing into the spinal cord as its cells differentiate into specific types of neurons [5] .
One crucial set of transcription factors is the 12-member LIM class, which is involved in determining where axons leave the neural tube [6] . In mice, a spinal motor neuron expressing the LIM transcription factors Lhx3 and Lhx4 exits ventrally, while neurons lacking Lhx3 and Lhx4 exit from a more dorsal location. Deficiencies in Lhx3 and Lhx4 in a ventral motor neuron shift the axon’s exit point more dorsally. Since the exit point plays a major role in determining the direction in which the axon goes, this shift causes the axon to innervate an incorrect region and prevents it from reaching its intended target [6] .
After the axon has exited the neural tube, it converges with other motor neuron axons in the ventral root and traverses through the front part of a somite, a structure directly adjacent to the neural tube that segments all of the incoming axons [7] . At the tip of the axon lies a growth cone, a structure containing numerous receptors that bind to guidance factors. Various guidance factors exist outside of the neural tube and these, as their name implies, maneuver the growth cones towards a particular target. In the arm, ephrins are one well-known class of guidance factors. Ephrins are proteins that have a specific ephrin receptor located in the growth cone of axons. When the motor neuron axon arrives at the base of the forelimb, it can innervate either dorsal or ventral muscles. Since our motor neuron of focus innervates the bicep, it will travel ventrally. When the growth cone of our neuron reaches the limb, the dorsal forelimb releases guidance factors including EphrinB. When EphrinB binds to its receptor on the motor neuron growth cone, it will repel the axon towards the ventral forelimb where the bicep is developing [7] . EphrinB acts like a magnet in this scenario, repelling the growth cone away from dorsal muscles since both have similar magnetic orientations. Other guidance factors can do the opposite by attracting the growth cone in the appropriate direction.
Amazingly, axonal guidance is conserved even if the motor neuron is shifted from its original position within the spinal cord. For example, in chick embryos, sections of the spinal cord have been moved to different locations in transplantation studies [8] . When the part of the spinal cord containing the motor neuron for the forelimb is moved to locations for motor neurons in the chest, the motor neuron will still send its axon over an even longer distance to reach the arm. When transplanted to even greater distances, such as to the part of the spinal cord containing neurons for the legs, arm motor neurons will still manage to correctly innervate the arm. The axonal growth cone on a specific motor neuron contains the same receptors for guidance factors despite the neuron’s shift in location, allowing for the same guidance factors to direct the axon to its correct place [8] .
Synapse Formation at the Neuromuscular Junction
Once the axon of our motor neuron reaches the bicep, it sends out multiple projections to different muscle fibers called myotubules, somewhat resembling the way our hands have multiple fingers that can each press individual letters on a keyboard [9] . This occurs in all developing motor neurons, and muscle fibers will initially have input from multiple neurons. In order for proper muscle function to occur, each muscle fiber must be innervated by exactly one motor neuron, though a single motor neuron can innervate multiple muscle fibers. It would be inefficient to have two neurons innervating the same muscle fiber, much like having two fingers press the same letter on the keyboard at the same time. If a muscle fiber is contracted by one motor neuron and another motor neuron signals the fiber to contract, it fails to respond correctly to the second signal. When the growth cone contacts a myotubule, it immediately establishes a premature synapse where acetylcholine, a neurotransmitter, is released from the axon onto the myotubule. Since this synapse is in its infancy, the myotubule does not have a sufficient number of acetylcholine receptors and responds inefficiently to the neuron. The absence of various presynaptic structures such as vesicle pools also results in the neuron releasing fewer amounts of acetylcholine than will eventually be needed for proper muscle contraction [9] .
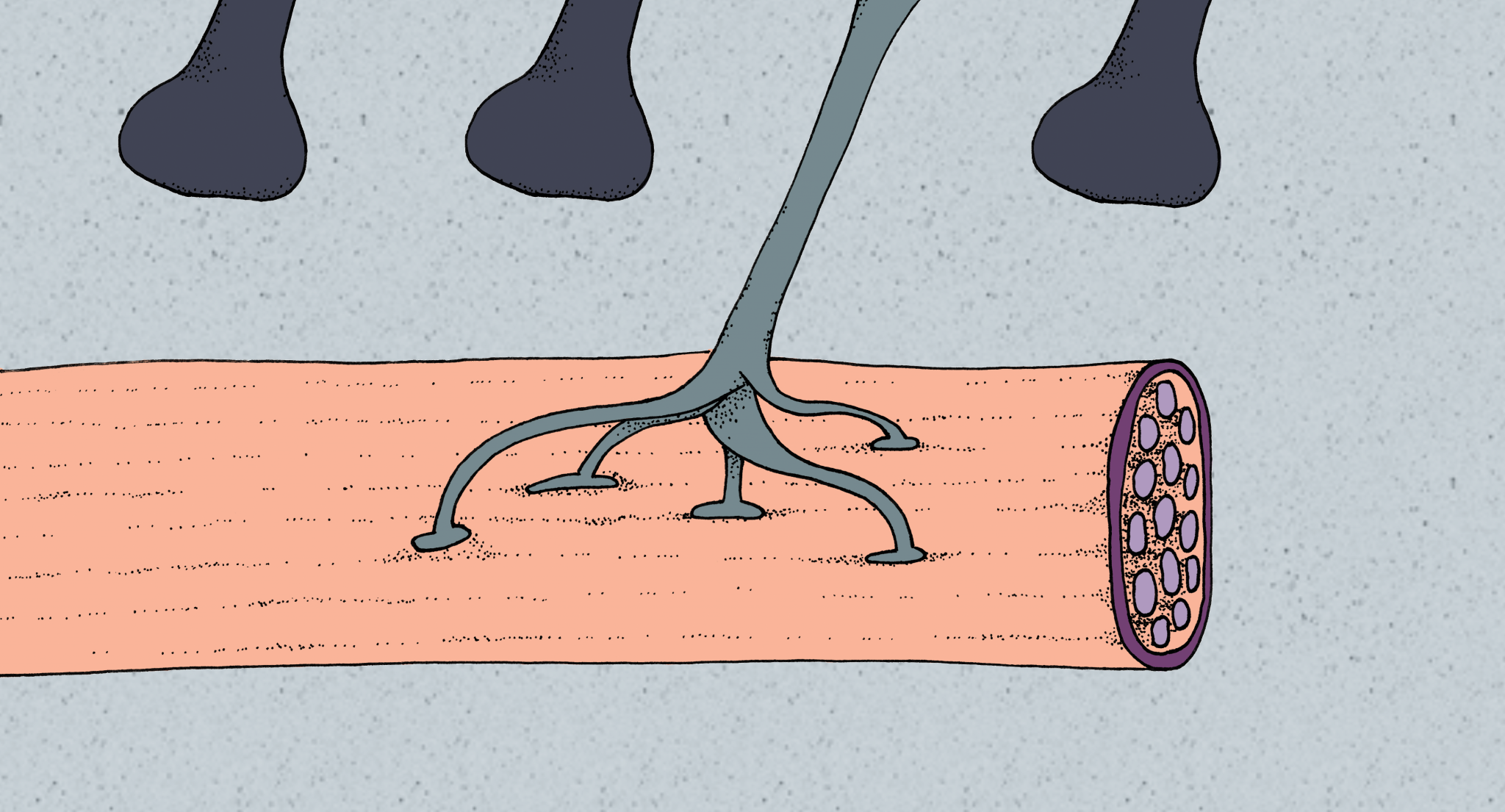
As the connection between the myotubule and neuron matures over time, the synapse can be strengthened or weakened [1] . One signal called agrin is released from the motor neuron and clusters acetylcholine receptors on the myotubule at the synapse. Due to the increased density of receptors, acetylcholine released from the motor neuron has a higher probability of eliciting depolarization, or an excitatory response, in the myotubule. Once depolarizations start to occur, the myotubule undergoes synaptic elimination to ensure only one motor neuron innervates the fiber. Elimination may occur due to the release of a synaptotrophin, a reward signal, from the myotubule. The current hypothesis is that axons receiving more synaptotrophin strengthen their synapses and starve other axons of the signal. Synaptotrophins are like food, as axons need to consume them in order to maintain a synaptic connection and grow, while those without them go hungry and retreat from the myotubule. Another proposed mechanism is the idea of a punishment signal called a synaptotoxin. Axons that elicit depolarizations in the myotubule do not uptake as much synaptotoxin as other axons, which accumulate higher concentrations of synaptotoxin over time. Using the same food analogy, synaptotoxins are expired food, making axons extremely sick if they have too much and forcing their retraction. Following the end of synaptic elimination, our motor neuron will successfully innervate a myotubule in the bicep and initiate contractions after further development [1] .
Conclusion
Once developed, the spinal motor neuron can relay activity from the brain as well as sensory structures to produce responses in the myotubules of the bicep. Other motor neurons undergo the same general developmental processes as our spinal motor neuron, completing the motor pathways to the rest of the muscles in the human body. Fortunately, neurodevelopmental disorders involving motor neurons are extremely rare; the vast majority of people are able to correctly coordinate muscle contraction throughout the entire body, allowing for the execution of necessary movements as early as gestation. Movement is remarkably complex, so it is no surprise that the development of the structures required for movement is similarly complex.
- Gilbert, SF. (2013). Developmental Biology: 10th Edition. Sunderland, MA: Sinauer Associates.
- Walløe, S., Pakkenberg, B., & Fabricius, K. (2014). Stereological estimation of total cell numbers in the human cerebral and cerebellar cortex. Front Hum Neurosci, 8. doi:10.3389/fnhum.2014.00508
- Stiles, J., & Jernigan, T. L. (2010). The Basics of Brain Development. Neuropsychol Rev, 20(4), 327-348. doi:10.1007/s11065-010-9148-4
- Briscoe, J., Pierani, A., Jessell, T. M., & Ericson, J. (2000). A Homeodomain Protein Code Specifies Progenitor Cell Identity and Neuronal Fate in the Ventral Neural Tube. Cell, 101(4), 435-445. doi:http://dx.doi.org/10.1016/S0092-8674(00)80853-3
- Philippidou, P., & Dasen, J. S. (2013). Hox Genes: Choreographers in Neural Development, Architects of Circuit Organization. Neuron, 80(1). doi:10.1016/j.neuron.2013.09.020
- Sharma, K., Sheng, H. Z., Lettieri, K., Li, H., Karavanov, A., Potter, S., . . . Pfaff, S. L. (1998). LIM Homeodomain Factors Lhx3 and Lhx4 Assign Subtype Identities for Motor Neurons. Cell, 95(6), 817-828. doi:http://dx.doi.org/10.1016/S0092-8674(00)81704-3
- Bonanomi, D., & Pfaff, S. L. (2010). Motor Axon Pathfinding. Cold Spring Harb Perspect Biol, 2(3). doi:10.1101/cshperspect.a001735
- Landmesser, L. T. (2001). The acquisition of motoneuron subtype identity and motor circuit formation. International Journal of Developmental Neuroscience, 19(2), 175-182. doi:http://dx.doi.org/10.1016/S0736-5748(00)00090-3
- Sanes, J. R., & Lichtman, J. W. (1999). Development of the Vertebrate Neuromuscular Junction. Annual Review of Neuroscience, 22, 389-442.