Introduction
Nineteen months after suffering a stroke, Patient “AC” was interviewed about how his limbs felt. He said about his right hand, “I can’t control it. It doesn’t listen to me…it betrays me…there is an animal in my arm; it got into my arm from this wound on my hand, do you see that? It is walking in here.” In another interview 5 months later, AC called his right hand “a fake hand”. When asked why, he explained that “sometimes at night it goes away; but then it leaps up and comes back, it climbs on my thigh and on the sheet” [1]. AC’s case is an example of brain injuries that reveal the precariousness of the human brain’s experience within the body. This is an example of the experience of embodiment, which refers to the sum of the brain’s many complex, fluid, and multilayered mental representations of the body [2]. These representations are often called body schema, and they deeply affect our perceptions and conceptions of the physical body they represent [3]. Proprioception, the sense of body movement and position, can be felt even while not moving. Amputees don’t have a limb to move or sense, but they frequently experience the sensation of a phantom limb in its place– the feeling that a limb is still there despite all evidence to the contrary. Such cases show that there are many different processes responsible for different ways in which we experience our bodies. As illustrated by AC, body schemas are flexible and can become disrupted by abnormal processing of sensory information in the brain, which in turn shifts our sense of embodiment out of alignment with our physical body. Furthermore, many of these processes rely on and share information with each other, which means that obstructions to any of them can have surprising domino-like consequences that produce experiences like AC’s: dream-like, impossible, and yet absolutely real [3].
Somatotopy
Our cortex is organized somatotopically: Cortical neurons are organized such that they create a map of the body. For example, the neurons that receive signals from your hands are physically adjacent to those that receive signals from your arms. Those arm neurons in turn are adjacent to neck neurons, and the neck neurons to face neurons. Interestingly, some points on the somatosensory cortex do not correspond with the expected body region based on anatomy. For example, cortical neurons innervating the thumb are next to neurons innervating the mouth [4]. The somatosensory cortex, which processes incoming sensory information such as touch and proprioception, and the motor cortex, which sends motor signals out to the body, are both organized in these somatotopic maps [5]. Interestingly, our brains are split into two hemispheres and the wiring of our hemispheres to our bodies is contralateral – in almost every case, the left side of the brain controls the right side of the body and vice versa [6].
Representations of these maps have earned themselves the name homunculi, from the Latin word meaning little man, for the way that they represent the body in comically out-of-proportion yet still anatomically-feasible ways. The homunculus' proportions are heavily distorted because they aren’t scaled according to the physical proportions of the body [7]. The greater the sensitivity and fine motor coordination of a part of the body, the greater the cortical space allotted in the somatosensory and motor cortices, respectively. For example, since a large portion of the somatosensory cortex is dedicated to signals sent from the fingertips, the fingertips on the homunculus are disproportionately large [7].
While the term ‘map' implies a perhaps unchanging landscape, these maps are far from static, as has been well demonstrated in people with Phantom Limb Syndrome.The neurons formerly responsible for an amputated limb in the somatotopic map may begin, post-amputation, to adopt responsibility for a non-amputated part of the body [8]. In an example of patients whose hands had been amputated, the part of the primary motor cortex that was responsible for their hand before it was amputated now lit up when they performed a lip-pursing action, suggesting that the brain had expanded the mouth area of the somatotopic map to include the neurons formerly associated with the now-missing hand [8]. These observations reflect neuroplasticity, the ability of the brain to adapt in response to environmental changes, such as brain damage, to reduce the negative impact of the change [9].
Another study trained arm amputees to move their phantom wrist in an anatomically impossible way [3]. Those who were successful reported that their success came at the same time as a self-reported profound change to the type of joint in their wrist, because the new joint made the motion anatomically possible. One patient, whose new phantom joint was of the planar style, described stiffness and fatigue in the phantom wrist and arm if he practiced the imaginary movement too much. Participants’ reports of this change in the internal anatomy of their phantom wrists was strongly corroborated by their performance on a motor imagery test, where participants sat with their hands in certain orientations and quickly answered questions when presented with images of hands rotated in various ways. The accuracy of their responses has been demonstrated as a solid way to measure the participants’ internal body schema of their hands. These tests allowed the researchers to empirically confirm the change, indicating the participants’ brains considered the nonexistent joint to actually exist [3].
The basis of embodiment
By mapping how our brains and bodies communicate, the somatosensory and primary motor cortices make crucial contributions to our experience of embodiment. However, they don’t account for all of it. We can be sure a signal is coming from a body, but how can we be sure it’s coming from our body?
It turns out we can’t—at least not definitively. Instead, our brains synthesize incoming multimodal sensorimotor information to make guesses about the different ways in which our bodies are present [10]. The simplest kind of presence is the existence of a body at all. Beyond that, ownership over that body and our agency to move it is established [10]. Due to the overlap of several distinct stimuli, when neural processing goes wrong, the prediction fails—sometimes in very strange ways [10].
If you’ve ever taken off a watch after wearing it for a prolonged period of time, you might be familiar with the lingering ghostly sensation that the watch is still physically there, as if it were a part of your body. For most of us, small hauntings like these are the most we will ever experience of our embodiments’ failed predictions. But in a testament to the fragility of our perception of our physical selves, more intense failures of embodiment can be induced in healthy individuals in a laboratory setting [10].
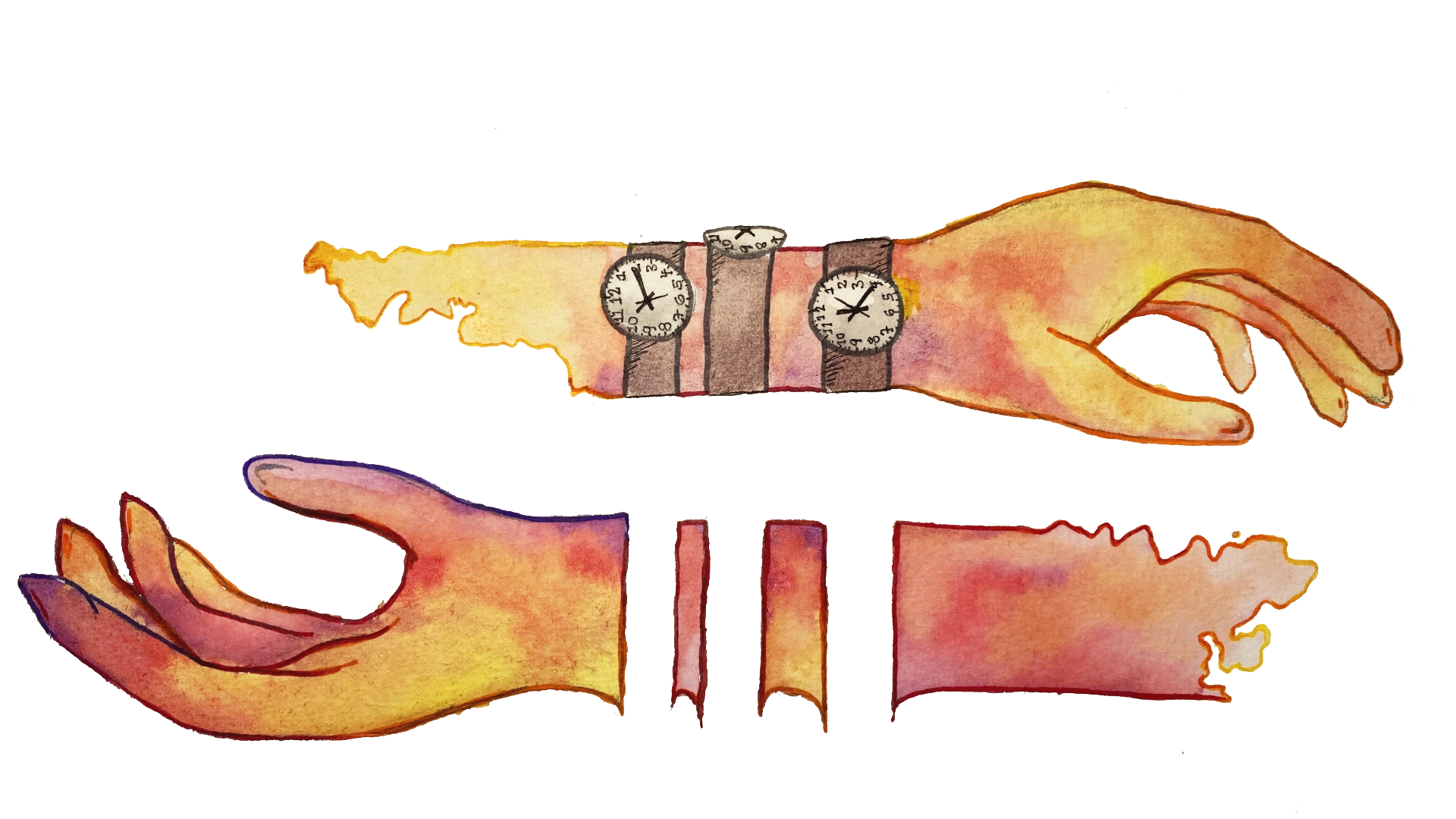
Rubber hand illusion (RHI)
In 1998, two researchers from Carnegie Mellon demonstrated a phenomenon that continues to be the subject of much neuroscientific research today: The rubber hand illusion [11]. Participants were seated behind a divider with a realistic rubber model of an arm in front of them. They could only see the fake arm, not their own arms. They then watched as the researchers touched the fake arm with paintbrushes, while simultaneously stroking the participants’ hidden real arm [11].
In a questionnaire conducted immediately after the experiment, almost all of the participants consistently answered that they agreed strongly with the statements “It seemed as though the touch I felt was caused by the paintbrush touching the rubber hand” and “I felt as if the rubber hand were my hand” [11]. These reports suggest the brain believed the fake arm to be their own, transferring the sensation of touch from the participants’ real arms to the fake arm.
The researchers also tested the participants’ proprioception in the dark. They found that the longer their hands had been stroked, the more participants demonstrated what is called “proprioceptive drift” towards the rubber hand, meaning that they were not able to accurately identify the location of their hand without being able to see it. When asked to slide their right hand under the table until they felt it was directly below the obscured left hand resting on top of the table, participants in whom the Rubber Hand Illusion (RHI) had just been induced performed the task less accurately. The direction of their margin of error was offset in the direction of the rubber hand, suggesting that their brains thought their right hand was the rubber hand [11]. Interestingly, slower stroking speeds seem to increase the effects of the RHI [12]. Slower stroking speeds, between 1 and 10 cm/s and most optimally at 3 cm/s, activate a group of nerves that respond to non-painful and slow touch [13].
A similar study tried controlling the experiment with a group that received no stroking and only looked at the hand. It found that while participants did not consciously think the rubber hand was their own, they still unconsciously produced the same proprioceptive drift as those whose hands were stroked during the RHI [14]. Both studies show how easily visual and sensory information can shift where the brain believes its own body to be, resulting in lower performance on proprioceptive tasks and the confusion of a fake limb for a real one.
RHI and the motor cortex
Further variations on the RHI investigated the role of the primary motor cortex on the illusion. Using a technique called low-frequency repetitive transcranial magnetic stimulation (rTMS), researchers applied inhibitory stimuli to the motor cortices of study participants in the experimental group before inducing the RHI [15]. Control groups included those who received rTMS inhibition on the area of the motor cortex that was responsible for the unobscured hand (the one not being replaced by a fake hand) and a placebo rTMS. Researchers found that participants had an easier time accepting the fake hand as their own after the primary motor cortex was inhibited. Practically, these results support the hypothesis that your ability to move your body contributes to your sense of ownership and embodiment within it [15].
The RHI’s effect of shifting the hand part of the body schema from the real hand to the rubber one means that the embodiment of the rubber hand is accompanied by disembodiment of the real (obscured) hand. This disembodiment does not just manifest as a subjective psychological experience; it is also physiological. Another study used an excitatory form of TMS to stimulate the hand part of the primary motor cortex on the hemisphere contralateral to the obscured hand using only a single pulse at a time [16]. When the motor cortex is stimulated, it sends an electrical signal down the descending motor pathways to the appropriate muscle that needs to be moved. These signals are known as motor evoked potentials (MEPs). The researchers measured the amplitude of MEPs generated in response to the TMS pulse administered to participants in the experimental RHI group and the control group, which received asynchronous tactile stimulation. The results paint a compelling picture of the relationship between movement and embodiment: MEPs sent by the motor cortex to the obscured hand muscles of experimental group participants who had RHI induced were significantly lower than those in control groups. In other words, the brain, under the effects of the RHI, was less capable of sending the electrical signals needed to make its disembodied hand move [16]. This doesn’t mean that the RHI paralyzes the disembodied hand. But there appears to be a cost to disembodiment, the effects of which create an experience of a hand that is less “ready” to be moved [16].
This evidence provides a part of the physiological basis for the psychological disembodiment experience of the RHI [16]. The experience of disembodiment is reflected in changes to the way the motor cortex fires. Whether decreased ownership of the body induced by the RHI manifests physically as impaired movement capability is not yet clear, but the research so far suggests that it doesn’t: When experiencing the effects of the RHI, you can still move your real hand just fine [17]. These findings together demonstrate that the primary motor cortex is important for embodiment, and that there appears to be a mutual relationship between the brain’s body schema and the brain’s ability to control and experience the physical body.
Embodiment and the integration of stimuli
Our brains are used to experiencing several incoming stimuli at once. The RHI works by disrupting these consistencies because our experience of embodiment arises in part from the integration of multimodal sensory information. Individual types of sensory data flow from all over the brain into key processing confluences. Neuroimaging techniques have identified some of these confluences in the ventral premotor cortex (vMPc), the posterior parietal cortex (PPc), and the temporal-parietal junction (TPJ) [18,19]. The vMPc and PPc work closely together to establish body ownership through primarily visual and tactile sensing, and are directly activated during the RHI [18]. The TPJ also integrates multimodal sensory information, but unlike the vMPc and PPc, it is heavily involved in sensory data that provides information about the body relative to itself and to the space around it. The TPJ plays key roles in body location, rather than ownership. The RHI has given researchers insight into the roles of the vMPc and PPc, but the TPJ as another site of embodiment processing is only the tip of the iceberg of the temporal and parietal contributions to embodiment [18,19].
Disorders of embodiment
The roles of top-down processing and the temporal and parietal lobes in producing body schema are slowly being puzzled out. Neuroimaging the brains of patients with various disorders of disembodiment allows researchers to find patterns in the locations of neural lesions they find and to identify the patient’s particular symptoms.
Asomatognosia is a broad descriptor of a cluster of neurological conditions in which parts of the patient’s body fade or disappear from their awareness. This loss of awareness may be constrained only to a loss of agency and/or ownership, or it may mean that the affected portion of the body feels like it does not exist at all, as if it had been amputated [20]. The general consensus is that asomatognosia specifically refers to a presentation of the phenomenon constrained only to one side of the body after a brain lesion to the opposite hemisphere. When asomatognosia presents more mildly, it is usually the result of a smaller lesion mostly confined to the parietal lobe [21]. In some cases, the patients regain awareness of the affected part of their body as soon as they look at it. Although the brain is improperly integrating somasthetic, or “body feeling,” information about the limb, it is still able to make use of the visual stimulus demonstrating evidence of the limb in order to project a correct body schema [21].
However, more extensive brain lesions across multiple lobes, particularly when some of the lesions are on the temporal lobe, are likely to produce a more severe kind of asomatognosia called somatoparaphrenia [22]. Somatoparaphrenia is characterized by an additional symptom in which patients recall false memories about their limbs. This was evident in the case of Patient AC, who described his hand almost as having a mind of its own, coming and going as it pleased [1]. These types of memory errors are called confabulations. They help the patient grapple with the existence of their limb in the face of inconsistent or absent information from their brain about it. In one case, a stroke patient’s brain attempted to reconcile the dissonance of being able to see her left hand while feeling that it wasn’t hers by recalling that it was “a hand that was left on the subway and they brought it here and they put it on me” [21]. Patients also frequently assign ownership of their limbs to family members, nurses, and other people who are frequently around them. Inversely, patients may believe that the limb of someone else—usually, someone in the room that they can see—is actually their own [20].
Another kind of more severe presentation is visual asomatognosia, also called somatoscopy, in which patients’ brains explain away the visual stimuli not through misattribution but instead through total non-identification. Someone with visual asomatognosia of their left arm looks down at the arm and just sees a void—either literally nothing at all, or they believe they can see, for example, the table underneath the place their arm rests [23].
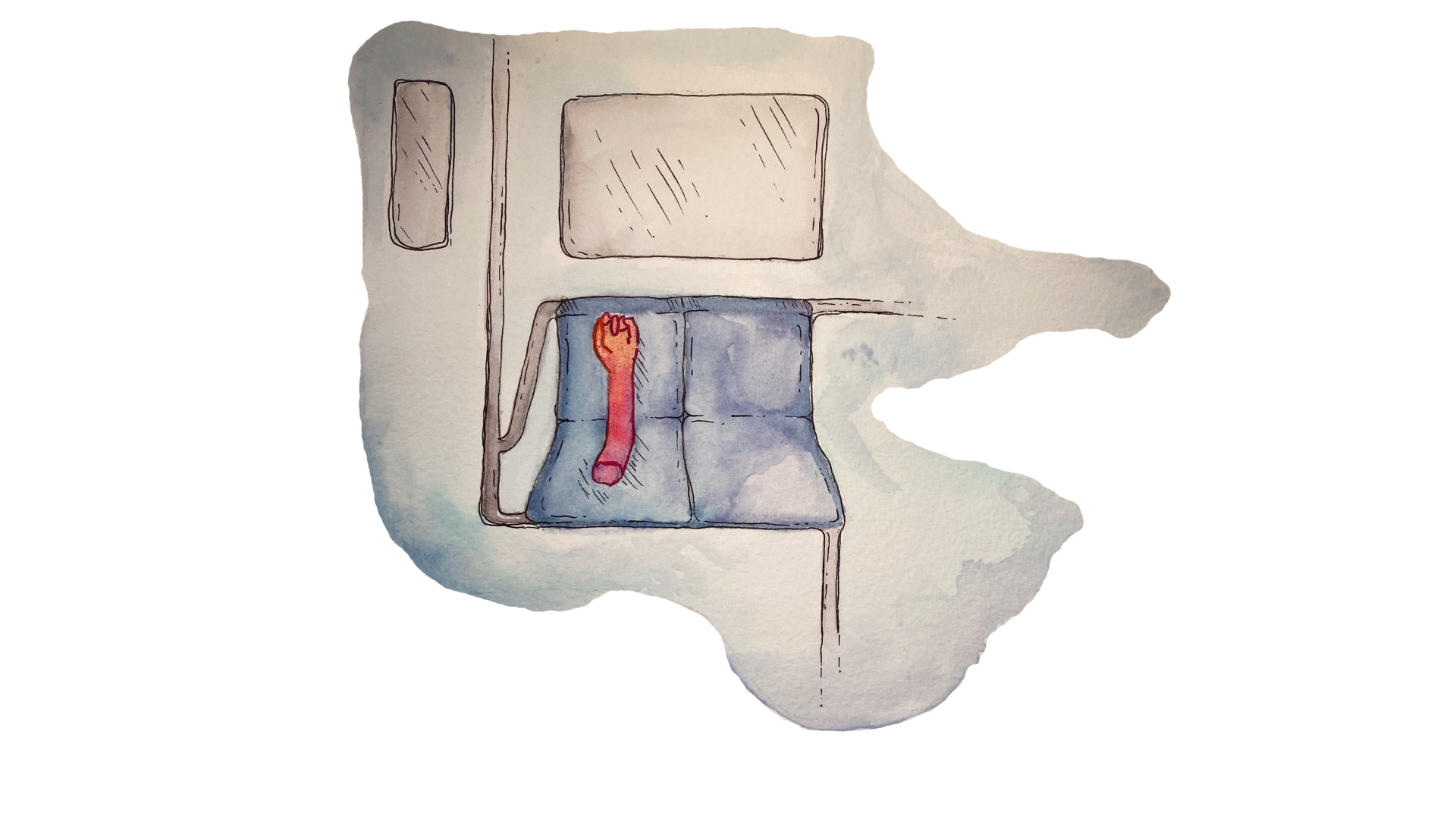
The RHI studies provide insight into the mechanism behind such disorders. Regions integrating different types of sensory input are of particular interest to those seeking to better understand abnormal embodiment. Future research should continue to merge both qualitative findings from patients suffering from a somatosensory disorder and also investigate how prosthetics or virtual reality may help relieve some of the symptoms [24].
Conclusion
Embodiment appears deceptively intuitive, so immediate and true there is no room for question: The sight of the body, the shape of the body, the sense of the body, and therefore: the body. But our brains are bright and impatient. We take needless shortcuts through our bodies so that we have already arrived when the physical realities of our bodies undergo the construction and destruction they are so terribly vulnerable to. Like cognition, corporeality is made efficient, adaptable, and resilient. To move is to fire the circuits made for moving and fulfill a capacity for motion. Sight is actual information absorbed into us, taking a different form once inside the brain but remaining information nonetheless. When the sense of ownership of a limb is damaged in a stroke, as was the case with patient AC, the eyes that are so used to holding the sight of that limb are thrown into a moment of crisis. The brain responds to the lack of stimuli by filling in the blank space where it can, with the sight of a loved one's arm or the sight of the table beneath the arm. In particular, the way that AC’s brain “filled in” missing information led to the development of his somatoparaphrenia. The brain relies on the coordination of several neural and sensory processes in order to produce an accurate representation of one’s body in relation to the world. This sense of embodiment can be thrown off by many disturbances, producing an alternative and often confusing reality for those living with the damage.
References
- Cogliano R., Crisci C., Conson M., Grossi D., Trojano L. Chronic somatoparaphrenia: A follow-up study on two clinical cases. Cortex, 48(6), 2012, 758-767. https://doi.org/10.1016/j.cortex.2011.08.008
- Golaszewski, S., Frey, V., Thomschewski, A., Sebastianelli, L., Versace, V., Saltuari, L., Trinka, E., & Nardone, R. (2021). Neural mechanisms underlying the Rubber Hand Illusion: A systematic review of related neurophysiological studies. Brain and behavior, 11(8), e02124. https://doi.org/10.1002/brb3.2124
- Moseley, G. L., & Brugger, P. (2009). Interdependence of movement and anatomy persists when amputees learn a physiologically impossible movement of their phantom limb. Proceedings of the National Academy of Sciences of the United States of America, 106(44), 18798–18802. https://doi.org/10.1073/pnas.0907151106
- Harding-Forrester, S., & Feldman, D. E. (2018). Somatosensory maps. Handbook of clinical neurology, 151, 73–102. https://doi.org/10.1016/B978-0-444-63622-5.00004-8
- Andersen, R. A., & Aflalo, T. (2022). Preserved cortical somatotopic and motor representations in tetraplegic humans. Current opinion in neurobiology, 74, 102547. https://doi.org/10.1016/j.conb.2022.102547
- Güntürkün, O., Ströckens, F., & Ocklenburg, S. (2020). Brain Lateralization: A Comparative Perspective. Physiological reviews, 100(3), 1019–1063. https://doi.org/10.1152/physrev.00006.2019
- Nguyen JD, Duong H. (2022). Neurosurgery, Sensory Homunculus. StatPearls Publishing. https://www.ncbi.nlm.nih.gov/books/NBK549841/
- MacIver, K., Lloyd, D. M., Kelly, S., Roberts, N., & Nurmikko, T. (2008). Phantom limb pain, cortical reorganization and the therapeutic effect of mental imagery. Brain : a journal of neurology, 131(Pt 8), 2181–2191. https://doi.org/10.1093/brain/awn124
- Gulyaeva N. V. (2017). Molecular Mechanisms of Neuroplasticity: An Expanding Universe. Biochemistry. Biokhimiia, 82(3), 237–242. https://doi.org/10.1134/S0006297917030014
- Tekgün, E., & Erdeniz, B. (2022). Contributions of Body-Orientation to Mental Ball Dropping Task During Out-of-Body Experiences. Frontiers in integrative neuroscience, 15, 781935. https://doi.org/10.3389/fnint.2021.781935
- Botvinick, M., Cohen, J. (1998). Rubber hands ‘feel’ touch that eyes see. Nature 391, 756. https://doi.org/10.1038/35784
- van Stralen H., van Zandvoort M., Hoppenbrouwers S., Vissers L., Kappelle L., Dijkerman L. (2014). Affective touch modulates the rubber hand illusion, Cognition, 131(1), 147-158. https://doi.org/10.1016/j.cognition.2013.11.020.
- Pawling, R., Cannon, P. R., McGlone, F. P., & Walker, S. C. (2017). C-tactile afferent stimulating touch carries a positive affective value. PloS one, 12(3), e0173457. https://doi.org/10.1371/journal.pone.0173457
- Rohde, M., Di Luca, M., & Ernst, M. O. (2011). The Rubber Hand Illusion: feeling of ownership and proprioceptive drift do not go hand in hand. PloS one, 6(6), e21659. https://doi.org/10.1371/journal.pone.0021659
- Fossataro, C., Bucchioni, G., D'Agata, F., Bruno, V., Morese, R., Krystkowiak, P., & Garbarini, F. (2018). Anxiety-dependent modulation of motor responses to pain expectancy. Social cognitive and affective neuroscience, 13(3), 321–330. https://doi.org/10.1093/scan/nsx146
- Della Gatta, F., Garbarini, F., Puglisi, G., Leonetti, A., Berti, A., & Borroni, P. (2016). Decreased motor cortex excitability mirrors own hand disembodiment during the rubber hand illusion. eLife, 5, e14972. https://doi.org/10.7554/eLife.14972
- Kammers, M. P., Verhagen, L., Dijkerman, H. C., Hogendoorn, H., De Vignemont, F., & Schutter, D. J. (2009). Is this hand for real? Attenuation of the rubber hand illusion by transcranial magnetic stimulation over the inferior parietal lobule. Journal of cognitive neuroscience, 21(7), 1311–1320. https://doi.org/10.1162/jocn.2009.21095
- Ehrsson, H. H., Holmes, N. P., & Passingham, R. E. (2005). Touching a rubber hand: feeling of body ownership is associated with activity in multisensory brain areas. The Journal of neuroscience : the official journal of the Society for Neuroscience, 25(45), 10564–10573. https://doi.org/10.1523/JNEUROSCI.0800-05.2005
- Blanke O, Arzy S. The Out-of-Body Experience: Disturbed Self-Processing at the Temporo-Parietal Junction. The Neuroscientist. 2005;11(1):16-24. https://doi.org10.1177/1073858404270885
- Jenkinson, P. M., & Preston, C. (2015). New reflections on agency and body ownership: The moving rubber hand illusion in the mirror. Consciousness and cognition, 33, 432–442. https://doi.org/10.1016/j.concog.2015.02.020
- Feinberg, T. E., Venneri, A., Simone, A. M., Fan, Y., & Northoff, G. (2010). The neuroanatomy of asomatognosia and somatoparaphrenia. Journal of neurology, neurosurgery, and psychiatry, 81(3), 276–281. https://doi.org/10.1136/jnnp.2009.188946
- Feinberg, T. E., & Venneri, A. (2014). Somatoparaphrenia: evolving theories and concepts. Cortex; a journal devoted to the study of the nervous system and behavior, 61, 74–80. https://doi.org/10.1016/j.cortex.2014.07.004
- Saetta, G., Zindel-Geisseler, O., Stauffacher, F., Serra, C., Vannuscorps, G., & Brugger, P. (2021). Asomatognosia: Structured Interview and Assessment of Visuomotor Imagery. Frontiers in psychology, 11, 544544. https://doi.org/10.3389/fpsyg.2020.544544
- Dieguez, S., & Lopez, C. (2017). The bodily self: Insights from clinical and experimental research. Annals of physical and rehabilitation medicine, 60(3), 198–207. https://doi.org/10.1016/j.rehab.2016.04.007