In 1976, French neuroscientist Marie-Germaine Bousser recruited hundreds of Parisians for an experiment to determine whether aspirin could prevent subsequent strokes in patients who had already had strokes. Amidst a flood of medical records and paperwork, the symptoms of a single patient enrolled in the study puzzled Bousser. This patient, a 50-year-old man, had a stroke despite the complete absence of common risk factors such as high blood pressure or heart disease [1][2]. After the conclusion of her successful aspirin study, Bousser’s interests turned immediately to the 50-year-old man. A scan of the man’s brain revealed small abnormalities and damage from multiple deep-lying subcortical strokes, valuable information that nonetheless did little to clarify the exact nature of the man’s disease. The man’s symptoms closely resembled those of Biswanger’s disease, a condition involving damage or degeneration of small blood vessels within the brain, but the absence of high blood pressure and the early onset of symptoms made Bousser hesitant to make this diagnosis. Instead, Bousser tracked the man’s disease over the next several years, noting the progressive development of muscle weakness, gait and balance difficulties, and dementia [1].
Still, the puzzling disease evaded diagnosis, and Bousser was unable to match its symptoms with known small vessel diseases of the brain. The turning point came when two of the man’s children, both in their 30s, came to Bousser with similar symptoms [1]. Using the brain-imaging technique magnetic resonance imaging (MRI), Bousser observed the same tissue damage and white matter changes in the children. Realizing that the disease was likely hereditary, Bousser, collaborating with neuroscientist Elisabeth Tournier-Lasserve, gathered 57 of the man’s closest relatives to conduct an exhaustive clinical study. The two discovered that half of the family members exhibited similar abnormalities under MRI, demonstrating a pattern of dominant genetic inheritance [3]. When Bousser and Tournier-Laserve were unable to match the disease to any previously known hereditary causes of stroke, they realized that they had discovered a new disease, now known as CADASIL.
CADASIL stands for Cerebral Autosomal Dominant Arteriopathy with Subcortical Infarcts and Leukoencephalopathy. This name is a mouthful, but each term is important for the definition of the disease. “Cerebral” refers to the main target of the disease, the brain. “Autosomal dominant” describes the genetic nature of the disease and its dominant inheritance pattern. “Arteriopathy” means that the disease affects the arteries, specifically those that supply blood to the brain. “Subcortical infarcts” clarifies that the disease affects small vessels deep below the surface of the brain and that the resulting decrease in blood flow causes damage in nearby brain structures like the basal ganglia and thalamus [4]. Finally, “leukoencephalopathy” means that there is damage to white matter, the axons that form connections between neurons and different parts of the brain. These terms describe the way CADASIL is inherited and how it affects the brain, however, the disease also causes several distinct symptoms. During her initial study of the disease, Bousser identified migraines, strokes, mood disturbances, cognitive decline, and dementia as the characteristic symptoms of CADASIL [3].
In the following years, CADASIL continued to be a topic of interest for Bousser and other scientists with whom she has collaborated. By 1996, mutations on the NOTCH3 gene on chromosome 19 were identified to be a cause of CADASIL, allowing for identification of the disease via genetic tests [5]. Over the last 25 years, scientists are slowly starting to understand the connection between NOTCH3 and blood vessels that cause CADASIL. Studies continue into the exact biological mechanisms behind the disease. CADASIL is of particular interest to neuroscientists because of its genetic nature. Since CADASIL is caused by simple mutations in a single gene, it can be both replicated in animal models and identified prior to the onset of its characteristic symptoms with genetic testing. As a result, neuroscientists now view CADASIL as a model not only for other diseases that affect the small vessels of the brain, but also dementia, migraine, and stroke [1]. Almost 50 years after M.G. Bousser first began to investigate it, ongoing research is finally elucidating CADASIL's mysterious mechanisms, paving the way for future therapeutic trials in not only CADASIL but other cerebral small vessel diseases as well.
The Genetic Nature of CADASIL and Animal Models
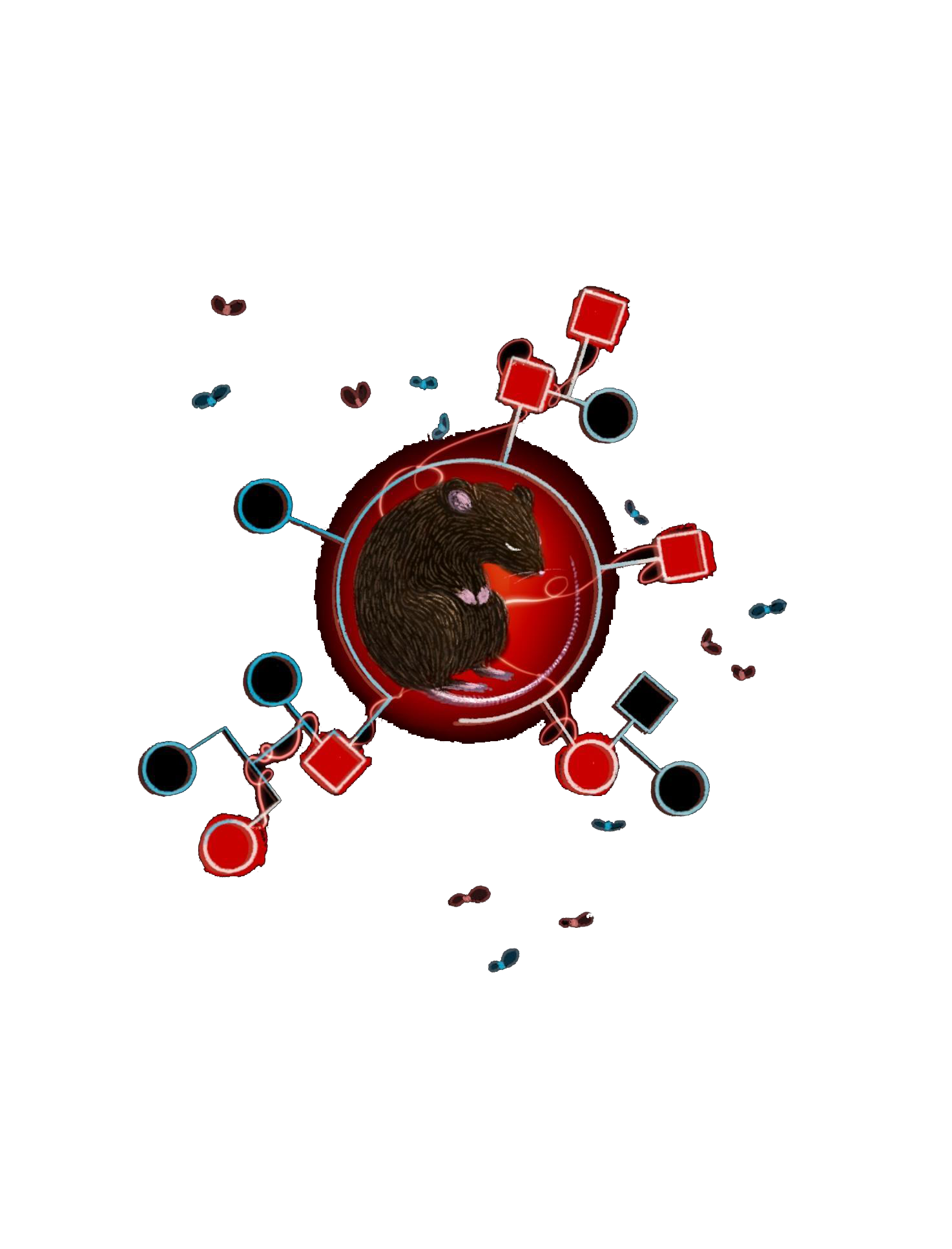
Following the initial discovery of the disease by Bousser and Tournier-Lasserve, research into CADASIL can be split into two distinct but intertwined categories. The first of these is purely scientific, focused on improving our academic understanding of CADASIL. The second category is more translational, hoping to uncover therapies for both CADASIL and other similar cerebrovascular diseases. Ultimately, the goal is that a greater understanding of the mechanisms of the disease will help to make new discoveries for the treatment of CADASIL.
For most diseases, the linking of these two categories is difficult. In some cases, scientists make progress in elucidating the mechanisms of the disease but have difficulty applying those findings to real treatments. One example is a neurodegenerative disease called amyotrophic lateral sclerosis (ALS), where recent improvements in the understanding of its pathophysiology have yielded little in the form of usable treatments [6]. On the other hand, treatments can also be found without a cohesive understanding of the disease. Despite the existence of a litany of antidepressant drugs, there is little consensus on the root causes of depression [7]. While these drugs are proven to be effective in the majority of patients, this lack of basic understanding means that the development of new and better drugs is difficult. Even though CADASIL research is still in its early stages, the precise reason why CADASIL is of such great interest is that it has a firm genetic basis. This genetic basis links the academic and clinical understanding of the disease together, making both types of research easier.
Since the 1970s, there have been several key genomic studies on CADASIL. In 1993, researchers examined data from the extended family of the first identified CADASIL patient. By comparing the pattern of CADASIL inheritance to the inheritance patterns of genes with a known location on the genome, scientists were able to map the disease to chromosome 19 [5]. This data allowed the researchers to identify 13 additional families, using the same technique to further narrow down the location of the disease [5]. In 1996, this research culminated in the identification of mutations in the NOTCH3 gene as the cause of CADASIL. This gene had previously not been known to exist in humans, despite its identification in fruit flies and mice. Further investigation has taken place on the exact mutations in the NOTCH3 gene that facilitate the development of CADASIL. In 1997, French geneticist Anne Joutel discovered that CADASIL was usually caused by mutations in specific regions (exons) of the gene that code for a region called the EGF-like domain in the NOTCH3 protein. This genetic finding suggested that CADASIL originates in some way from dysfunction in the EGF region of the NOTCH3 protein. Genomic studies continue to this day. In 2018, a collaborative study headed by the CADASIL Research Group in the Netherlands found that the variable severity of CADASIL is likely caused by slight differences in the location of the mutations in the NOTCH3 gene [8]. Studies like this one increase the number of potential therapeutic targets for scientists.
There are two main reasons why a genetic understanding of CADASIL is so beneficial to its general study. First, the ability to test for CADASIL before the appearance of outward symptoms is invaluable. Because scientists are able to identify individuals who will develop CADASIL, they can document the complete progression of the disease. Using MRIs, scientists have been able to elaborate on the clinical manifestations of the disease in both its early and late stages. Second, the understanding of the genetic nature of CADASIL aids in the development of animal models. Because studying diseases in humans is difficult and comes with greater ethical considerations, accurate replication of disease in animals is helpful to researchers in studying the underlying causes and mechanisms. In the case of CADASIL, early research was done using Drosophila fruit flies as a model. In flies, a condition called lethal-Abruptex, which interrupts wing growth, is caused by mutations in the EGF portion of the Notch protein [9]. When CADASIL was discovered to have a similar mechanism of action, scientists honed in on this Drosophila condition as a potential model for the study of CADASIL. Using Drosophila, researchers found evidence for the importance of NOTCH3 in the function of smooth muscle cells and tested gene therapy as a candidate for treatment by correcting the NOTCH3 mutation in Drosophila [9]. More recently, research on CADASIL has turned towards using mice models. Because mice are evolutionarily closer to humans than flies, they tend to make better models for human diseases. Using five different types of NOTCH3 mutant mice, researchers now have a better understanding of the physiological changes that result in the CADASIL phenotype [10]. While much of this research raises as many questions as it answers, a number of the discoveries that we will discuss in this article are built on the foundation of mouse models.
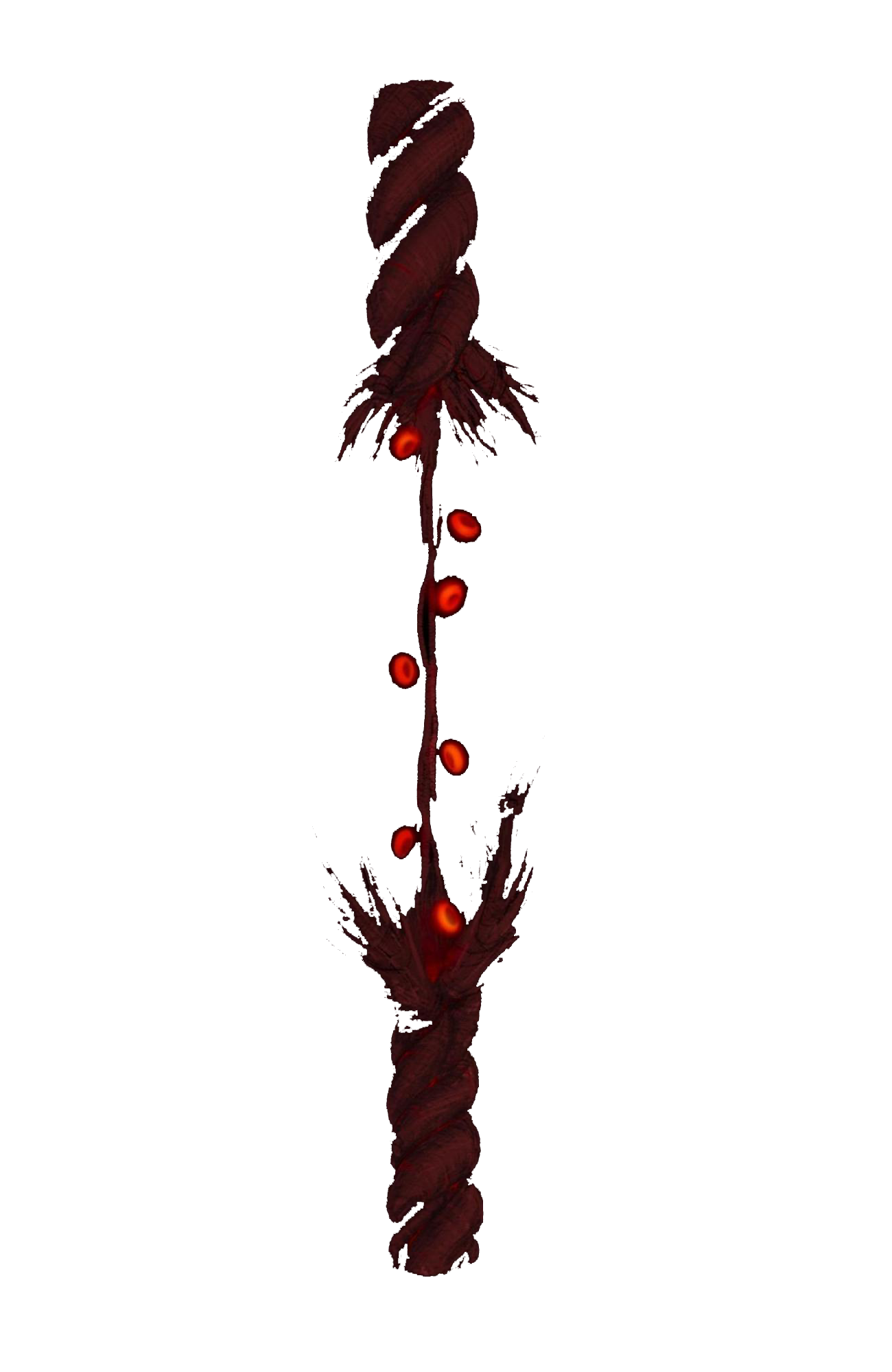
Insights Into the Biological Mechanisms of CADASIL
To understand CADASIL, we must first have a better understanding of the function of NOTCH3. The NOTCH3 gene provides instructions for making a receptor protein called NOTCH3 [11]. This type of protein has two ends, an extracellular domain that resides outside the cell membrane and an intracellular domain that is within the cell. When the extracellular domain of the NOTCH3 receptor protein binds with a ligand, the intracellular domain detaches and travels to the nucleus where it controls gene expression. NOTCH3 is primarily found in vascular smooth muscle cells (VSMCs), which are a major component of the wall of arteries. VSMCs maintain the integrity of arteries and aid in the control of cerebral blood flow. Because NOTCH3 mutations in CADASIL cause symptoms that are the result of blood vessel degeneration, it is evident that NOTCH3 has an important role in the sustained function of these VSMCs [11]. Still, the differences in NOTCH3 function that cause CADASIL are not very well understood. Even today, scientists do not have a mechanistic explanation that links NOTCH3 mutations with the pathology of small vessels in CADASIL. An understanding of this pathway is important because it could provide targets for potential therapeutics. As a result, most current CADASIL research is centered around finding the direct effects of NOTCH3 mutations, then connecting those effects to VSMC dysfunction.
Historically debated among researchers was whether CADASIL was the result of a gain-of-function mutation or a loss-of-function mutation. If NOTCH3 was subject to a gain-of-function mutation, the protein would either become more active or acquire a different function. If NOTCH3 was the result of a loss-of-function mutation, the protein would become less active. Because loss-of-function mutations are both more common and conveniently explain long-term cell degeneration, scientists initially hypothesized that NOTCH3 loss-of-function was the cause of CADASIL. The identification of a correlation between homozygous CADASIL patients and a more severe expression of the disease seemed to back up that theory [11]. If the mutation was loss-of-function, then heterozygotes would have improved NOTCH3 function compared to homozygotes. As a result, the finding that homozygotes have greater disease severity indicates that they have less NOTCH3 function, suggesting that the mutation is loss-of-function.
Recent evidence, however, strongly suggests that NOTCH3 undergoes a gain-of-function mutation. While investigation into human subjects supports this theory, the strongest evidence comes from studies using mice. In humans, CADASIL is always accompanied by the accumulation of deposits of NOTCH3 extracellular domain (Notch3ECD) in the small vessels and in aggregates called granular osmiophilic materials (GOMs). This aggregatory tendency also occurs in cells with the CADASIL genotype grown in the lab [12]. In mice where NOTCH3 is removed, some parts of the disease phenotype are observed, but the characteristic aggregation of Notch3ECD is not observed [13]. In mice genetically engineered to have the same NOTCH3 mutations as humans, both Notch3ECD aggregation and GOM formation are present [14]. As a whole, these studies suggest that NOTCH3 aggregation is a contributing factor to the development of CADASIL symptoms and not just a byproduct. This aggregation is unlikely to occur due to a loss-of-function, but rather as a result of changes in the binding of NOTCH3 that would be characteristic of gain-of-function. In summary, these studies indicate CADASIL is not the result of a disruption in NOTCHh3 signaling (loss-of-function), but rather the result of an unexpected function of Notch3ECD (gain-of-function) that occurs when it accumulates in the small vessels.
With strong evidence that Notch3ECD aggregation may be the cause of VSMC degeneration, CADASIL researchers are now hoping that an examination of Notch3ECD deposits can reveal a direct connection to CADASIL symptoms. In a seminal paper published in Brain in 2013, researchers in Anne Joutel’s lab kicked off this process [15]. Using a variety of complex biochemical techniques, these researchers were able to separate the Notch3ECD aggregates from other tissue, then identify two different proteins that were abnormally abundant in the sample. These two proteins, TIMP3 and VTN, are sequestered in the Notch3ECD aggregates, interacting in ways that further enhance the accumulation of the proteins. Building on these findings, the researchers examined mice and human patients with CADASIL, confirming the presence of elevated TIMP3 activity. Since these proteins are both functionally important for maintenance of the small vessels, disruption in their function could cause the development of problems in the small vessels, which would explain VSMC degeneration [15].
Since that study was published, scientists have found a number of different proteins that are sequestered in Notch3ECD deposits, including clusterin, decorin, biglycan, and APCS [14]. Although the exact role of all these proteins is still being researched, they present potential therapeutic targets. In practice, when TIMP3 and VTN levels (expression) are reduced in CADASIL mice, the disease symptoms are alleviated, and damage to white matter is reduced [16]. At the very least, this study shows that Notch3ECD deposits have some influence on CADASIL, and regulating the levels of TIMP3, VTN, and other proteins could have beneficial therapeutic effects. Ongoing studies on clusterin, decorin, and biglycan have the potential to strengthen this hypothesis.
Still, Notch3ECD deposits are not the be-all and end-all of CADASIL research. Scientists are increasingly studying secondary CADASIL effects, and their insights can provide other perspectives on the disease. In a 2017 paper, a group of Italian physicians did exactly that. Surveying the blood of patients, these scientists found that CADASIL individuals had higher levels of a protein called von Willebrand factor (vWF) and lower levels of a type of stem cell called endothelial progenitor cells (EPCs) [17]. Because this imbalance is found in humans with damage to their endothelial cells, the findings suggest that these endothelial cells are involved in CADASIL. Endothelial cells, which line the inside of all blood vessels, help sustain homeostasis in blood vessels and interact with VSMCs. The results of the study are significant because they suggest that the effect of Notch3ECD on VSMCs is not the sole cause of blood vessel dysfunction. Instead, both endothelial and VSMCs are significant, and the CADASIL genotype likely affects the interactions between the two types of cells. A 2018 paper by researchers at Newcastle University in the UK hints at even more subtleties to the biological relationships that govern CADASIL. These researchers found that astrocytes, a type of glial cell, undergo frequent cell death among CADASIL patients [18]. Astrocytes support endothelial cells and help to regulate cerebral blood flow, making them another logical suspect in the dysfunction of blood vessels that occurs in CADASIL. As a whole, these studies tell us only that CADASIL is the result of some combination of these different factors. Though new research seems to raise as many questions as it answers, each paper adds a piece of the puzzle and helps unveil the connections responsible for CADASIL.
Future Study and Application to other Diseases
Nearly a half-century after Bousser first identified CADASIL, researchers are still not particularly close to developing any form of pharmaceutical therapy. As of today, there are no ongoing clinical trials for CADASIL drugs, and the treatment of CADASIL patients is limited to management of their symptoms. Still, CADASIL researchers are optimistic that therapeutics are not too far off in the future. Recent progress in understanding the disease mechanism of CADASIL has had two main applications. First, these advances have given researchers new ideas for designing potential human trials. Second, these advances clarify how CADASIL functions as a model for other cerebral small vessel diseases.
With its accumulation in small vessels now understood, Notch3ECD has become a natural target for therapeutic development. In one 2018 study, this focus has already yielded successful results, albeit preliminary [19]. Amongst a collection of antibodies, researchers found an antibody that binds specifically to Notch3ECD aggregates in the blood vessels. When this antibody was administered to CADASIL mutant mice, they were protected against cerebrovascular dysfunction. The results of this study were not perfect; for instance, the researchers did not observe any difference in the appearance of brain lesions. The mechanism of the antibody is not understood [19], but the study still acts as a proof of concept for this avenue of CADASIL treatment, both in humans and mice.
Gaining a better understanding of the disease mechanisms of CADASIL has also led to the discovery of new biomarkers. Biomarkers are measurable items that indicate the status or progression of a disease. For CADASIL, one particularly promising biomarker is the protein neurofilament light chain (NfL). Neurofilaments are components of the cytoskeleton of neurons, and they appear in the bloodstream as a result of axonal damage [20]. In recent years, NfL has been used as a marker for amyotrophic lateral sclerosis (ALS), multiple sclerosis (MS), and Alzheimer’s disease [20]. In a 2018 study, researchers at the University of Basil found that the severity of symptoms in CADASIL patients was strongly correlated with levels of NfL in blood [21]. Biomarkers like NfL are essential to therapeutic development because they allow researchers to diagnose and track the progression of the disease, as well as measure therapeutic effectiveness. In the case of CADASIL, NfL could allow researchers to conduct therapeutic trials with smaller sample sizes and over a shorter period of time.
Lastly, improvements in the understanding of CADASIL make it a more effective model for the study of other diseases. Since the discovery of CADASIL, scientists have viewed it as a potential model for cerebral small vessel diseases (SVD), a group of diseases that commonly include symptoms like stroke and dementia [22]. This initial interest was due to the genetic nature of CADASIL, allowing it to be identified and studied early in the progression of the disease. Because small vessel diseases are usually not identified until they cause visible symptoms, CADASIL allows researchers to draw conclusions about how this group of diseases occurs in its earlier stages. However, scientists have been reluctant to generalize their discoveries from CADASIL because the mechanisms of CADASIL are not well understood. granular osmiophilic material (GOM) deposits, for example, are found exclusively in CADASIL patients. If GOMs are the sole determinants of CADASIL, then CADASIL is probably not a good model for other small vessel diseases. But as research into CADASIL continues, researchers have been able to connect CADASIL and SVDs with greater certainty. Features of CADASIL like vascular smooth muscle cell death, the loss of endothelial cells, and NOTCH3 receptor mutations have all been observed in SVDs [22]. These similarities indicate that the same pathways may contribute to both genetic and non-genetic SVDs. Still, CADASIL is not a perfect model. The question of GOM deposits being unique to CADASIL remains. As a whole, a more nuanced understanding of CADASIL clarifies both its advantages and disadvantages as a model, allowing it to be used with greater certainty.
Conclusion
In 2019, M.G. Bousser, Elizabeth Tournier-Lasserve, Anne Joutel, and Hugues Chabriat won the Brain Prize for their work in CADASIL research [23]. Awarded annually, the Brain Prize is essentially the Nobel Prize of neuroscience research and comes with a tidy sum of around $1.5 million. Although the 2019 Brain Prize reflects how important their pioneering work was, it also shows the growing awareness among the neuroscience community of the future potential of CADASIL as a model. While the first generation of CADASIL researchers was small in population, there are now thousands of scientists internationally working on CADASIL and hundreds of new research papers each year. Many of these scientists are using their expertise in different fields to bring a new perspective to CADASIL research. At the University of Washington, genome scientists are starting to use a new technique called multiplex assays of variant effects to create a more detailed map of how single nucleotide changes affect NOTCH3 [24]. Other scientists are using CRISPR/cas-9, a new gene-editing system. Research using CRISPR/cas-9 has already been helpful in clarifying how certain mutations affect NOTCH3 protein aggregation [25]. While the 2019 Brain Prize may be the culmination of the pioneering work of the initial group of French CADASIL researchers, CADASIL research as a whole is still in its early stages. As more research focus turns to CADASIL, who knows what the future will bring?
References
- Chabriat, H., Joutel, A., Tournier-Lasserve, E., & Bousser, M. G. (2020). CADASIL: yesterday, today, tomorrow. European journal of neurology, 27(8), 1588–1595. https://doi.org/10.1111/ene.14293
- Bousser, M. G., Eschwege, E., Haguenau, M., Lefaucconnier, J. M., Thibult, N., Touboul, D., & Touboul, P. J. (1983). "AICLA" controlled trial of aspirin and dipyridamole in the secondary prevention of athero-thrombotic cerebral ischemia. Stroke, 14(1), 5–14. https://doi.org/10.1161/01.str.14.1.5
- Tournier-Lasserve, E., Iba-Zizen, M. T., Romero, N., & Bousser, M. G. (1991). Autosomal dominant syndrome with strokelike episodes and leukoencephalopathy. Stroke, 22(10), 1297–1302. https://doi.org/10.1161/01.str.22.10.1297
- Kwon D. Y. (2016). Movement Disorders Following Cerebrovascular Lesions: Etiology, Treatment Options and Prognosis. Journal of movement disorders, 9(2), 63–70. https://doi.org/10.14802/jmd.16008
- Joutel, A., Corpechot, C., Ducros, A., Vahedi, K., Chabriat, H., Mouton, P., Alamowitch, S., Domenga, V., Cécillion, M., Marechal, E., Maciazek, J., Vayssiere, C., Cruaud, C., Cabanis, E. A., Ruchoux, M. M., Weissenbach, J., Bach, J. F., Bousser, M. G., & Tournier-Lasserve, E. (1996). Notch3 mutations in CADASIL, a hereditary adult-onset condition causing stroke and dementia. Nature, 383(6602), 707–710. https://doi.org/10.1038/383707a0
- van den Bos, M., Geevasinga, N., Higashihara, M., Menon, P., & Vucic, S. (2019). Pathophysiology and Diagnosis of ALS: Insights from Advances in Neurophysiological Techniques. International journal of molecular sciences, 20(11), 2818. https://doi.org/10.3390/ijms20112818
- Hasler G. (2010). Pathophysiology of depression: do we have any solid evidence of interest to clinicians?. World psychiatry : official journal of the World Psychiatric Association (WPA), 9(3), 155–161. https://doi.org/10.1002/j.2051-5545.2010.tb00298.x
- Rutten, J.W., Van Eijsden, B.J., Duering, M. et al. The effect of NOTCH3 pathogenic variant position on CADASIL disease severity: NOTCH3 EGFr 1–6 pathogenic variant are associated with a more severe phenotype and lower survival compared with EGFr 7–34 pathogenic variant. Genet Med 21, 676–682 (2019). https://doi.org/10.1038/s41436-018-0088-3
- Fryxell, K. J., Soderlund, M., & Jordan, T. V. (2001). An animal model for the molecular genetics of CADASIL. (Cerebral autosomal dominant arteriopathy with subcortical infarcts and leukoencephalopathy). Stroke, 32(1), 6–11. https://doi.org/10.1161/01.str.32.1.6
- Ayata C. (2010). CADASIL: experimental insights from animal models. Stroke, 41(10 Suppl), S129–S134. https://doi.org/10.1161/STROKEAHA.110.595207
- Tuominen, S., Juvonen, V., Amberla, K., Jolma, T., Rinne, J. O., Tuisku, S., Kurki, T., Marttila, R., Pöyhönen, M., Savontaus, M. L., Viitanen, M., & Kalimo, H. (2001). Phenotype of a homozygous CADASIL patient in comparison to 9 age-matched heterozygous patients with the same R133C Notch3 mutation. Stroke, 32(8), 1767–1774. https://doi.org/10.1161/01.str.32.8.1767
- Duering, M., Karpinska, A., Rosner, S., Hopfner, F., Zechmeister, M., Peters, N., Kremmer, E., Haffner, C., Giese, A., Dichgans, M., & Opherk, C. (2011). Co-aggregate formation of CADASIL-mutant NOTCH3: a single-particle analysis. Human molecular genetics, 20(16), 3256–3265. https://doi.org/10.1093/hmg/ddr237
- Henshall, T. L., Keller, A., He, L., Johansson, B. R., Wallgard, E., Raschperger, E., Mäe, M. A., Jin, S., Betsholtz, C., & Lendahl, U. (2015). Notch3 is necessary for blood vessel integrity in the central nervous system. Arteriosclerosis, thrombosis, and vascular biology, 35(2), 409–420. https://doi.org/10.1161/ATVBAHA.114.304849
- Zellner, A., Scharrer, E., Arzberger, T., Oka, C., Domenga-Denier, V., Joutel, A., Lichtenthaler, S. F., Müller, S. A., Dichgans, M., & Haffner, C. (2018). CADASIL brain vessels show a HTRA1 loss-of-function profile. Acta neuropathologica, 136(1), 111–125. https://doi.org/10.1007/s00401-018-1853-8
- Monet-Leprêtre, M., Haddad, I., Baron-Menguy, C., Fouillot-Panchal, M., Riani, M., Domenga-Denier, V., Dussaule, C., Cognat, E., Vinh, J., & Joutel, A. (2013). Abnormal recruitment of extracellular matrix proteins by excess Notch3 ECD: a new pathomechanism in CADASIL. Brain : a journal of neurology, 136(Pt 6), 1830–1845. https://doi.org/10.1093/brain/awt092
- Capone, C., Cognat, E., Ghezali, L., Baron-Menguy, C., Aubin, D., Mesnard, L., Stöhr, H., Domenga-Denier, V., Nelson, M. T., & Joutel, A. (2016). Reducing Timp3 or vitronectin ameliorates disease manifestations in CADASIL mice. Annals of neurology, 79(3), 387–403. https://doi.org/10.1002/ana.24573
- Pescini, F., Donnini, I., Cesari, F., Nannucci, S., Valenti, R., Rinnoci, V., Poggesi, A., Gori, A. M., Giusti, B., Rogolino, A., Carluccio, A., Bianchi, S., Dotti, M. T., Federico, A., Balestrino, M., Adriano, E., Abbate, R., Inzitari, D., & Pantoni, L. (2017). Circulating Biomarkers in Cerebral Autosomal Dominant Arteriopathy with Subcortical Infarcts and Leukoencephalopathy Patients. Journal of stroke and cerebrovascular diseases : the official journal of National Stroke Association, 26(4), 823–833. https://doi.org/10.1016/j.jstrokecerebrovasdis.2016.10.027
- Hase, Y., Chen, A., Bates, L. L., Craggs, L., Yamamoto, Y., Gemmell, E., Oakley, A. E., Korolchuk, V. I., & Kalaria, R. N. (2018). Severe white matter astrocytopathy in CADASIL. Brain pathology (Zurich, Switzerland), 28(6), 832–843. https://doi.org/10.1111/bpa.12621
- Ghezali, L., Capone, C., Baron-Menguy, C., Ratelade, J., Christensen, S., Østergaard Pedersen, L., Domenga-Denier, V., Pedersen, J. T., & Joutel, A. (2018). Notch3ECD immunotherapy improves cerebrovascular responses in CADASIL mice. Annals of neurology, 84(2), 246–259. https://doi.org/10.1002/ana.25284
- Cai, L., & Huang, J. (2018). Neurofilament light chain as a biological marker for multiple sclerosis: a meta-analysis study. Neuropsychiatric disease and treatment, 14, 2241–2254. https://doi.org/10.2147/NDT.S173280
- Duering, M., Konieczny, M. J., Tiedt, S., Baykara, E., Tuladhar, A. M., Leijsen, E. V., Lyrer, P., Engelter, S. T., Gesierich, B., Achmüller, M., Barro, C., Adam, R., Ewers, M., Dichgans, M., Kuhle, J., de Leeuw, F. E., & Peters, N. (2018). Serum Neurofilament Light Chain Levels Are Related to Small Vessel Disease Burden. Journal of stroke, 20(2), 228–238. https://doi.org/10.5853/jos.2017.02565
- Locatelli, M., Padovani, A., & Pezzini, A. (2020). Pathophysiological Mechanisms and Potential Therapeutic Targets in Cerebral Autosomal Dominant Arteriopathy With Subcortical Infarcts and Leukoencephalopathy (CADASIL). Frontiers in pharmacology, 11, 321. https://doi.org/10.3389/fphar.2020.00321
- The brain prize winners. Lundbeckfonden. (2019). Retrieved December 7, 2021, from https://lundbeckfonden.com/the-brain-prize/the-brain-prize-winners.
- Current research. CADASIL Eradication Project. (2019, December 27). Retrieved December 7, 2021, from https://www.notch3.org/current-research/.
- Gravesteijn, G., Dauwerse, J. G., Overzier, M., Brouwer, G., Hegeman, I., Mulder, A. A., Baas, F., Kruit, M. C., Terwindt, G. M., van Duinen, S. G., Jost, C. R., Aartsma-Rus, A., Lesnik Oberstein, S., & Rutten, J. W. (2020). Naturally occurring NOTCH3 exon skipping attenuates NOTCH3 protein aggregation and disease severity in CADASIL patients. Human molecular genetics, 29(11), 1853–1863. https://doi.org/10.1093/hmg/ddz28.