In the ocean, the form water will take at any given time may seem unpredictable to someone not paying attention, though there are patterns that can be identified and relied upon with some certainty. Towards the shore, for instance, water takes the form of smaller waves, and their crests appear closer together as they approach the sand. As you go deeper into the water, further away from the shore, the waves become less frequent. When you do notice a wave, it is often larger than those closer to shore, though smaller waves do still exist in the deeper waters.
In this way, the brain is similar to the ocean, as the brain’s neural activity can be visualized as waves similar to those at the beach, with the peaks indicating high neural activity and the dips indicating low neural activity [1]. Our brain waves change depending on whether we are awake or asleep, and the patterns in these waves can be used to identify which stage of sleep we are in [2].
Imagine yourself and your brain taking a nap at the beach. As you fall asleep, your brain begins to venture out into the water, first experiencing the small waves towards the shore. Later, as your sleep deepens and your brain ventures deeper into the water, it experiences more of the larger, less frequent waves found in these waters. Once the deepest stage of sleep is reached, the farthest you can be away from the shore, your brain does not stay there. Instead, it cycles through these various levels of sleep, which are divided into specific stages, until you wake up, almost as though it is swimming laps [2].
Sleep is one of the few universally performed acts among animals, and for humans the effects of a lack of sleep are often quickly noticed by those experiencing it. Sometimes, even if a person manages to get the recommended amount of sleep, they may still feel tired and lethargic the next day and may also experience some memory problems [3]. When this occurs, it is often a result of poor slow wave sleep (SWS), a stage of sleep that is important for sleep quality and strengthening memories. Researchers have hypothesized that this is due to the specific brain wave patterns that occur during this stage, which could be visualized as being similar to the deep ocean waves furthest from the shore [3]. With this example, poor SWS could be visualized as the ocean on a rainy day. The larger waves are interrupted by the ripples created as raindrops hit the water, so the waves do not appear as clean or synchronized.
As a result, scientists have been focusing on the deepest stage of sleep, known as slow wave sleep, and the changes our bodies experience during this specific period of time [4]. As scientists examine these processes and the mechanisms behind them, they have been able to gain insight into ways we can improve our sleep.
SWS occurs during a period called non-rapid eye movement sleep (NREM) [4]. NREM is divided into three stages categorized by different types of brain waves. Stage 1 is the stage we experience right after we fall asleep and is the closest to an awake state. Stage 2 occurs a few minutes after Stage 1 and marks the descent into deeper sleep states. Stage 3, also known as SWS, occurs right at the end of a sleep cycle and is the deepest sleep stage. Most SWS happens early in the night and takes up anywhere between 10-25% of our total sleep time. As the night progresses, the time devoted to SWS in each cycle decreases and is replaced by time spent in what is known as rapid-eye movement (REM) sleep [4]. Our brains cycle through these stages about four to five times a night, with each sleep cycle lasting anywhere between 90 to 110 minutes. In total, about 75% of sleep is spent in NREM, including SWS [2]. The longer we are awake, the more slow waves we experience during SWS, suggesting its importance in recovery and sleep quality [4].
Despite taking up a small portion of our sleep, SWS plays a crucial role in our sleep quality and may be responsible for the restorative properties of sleep [4]. Clinical studies have found that SWS is often affected in those with various sleep disorders, including insomnia and sleep apnea. Those who suffer from issues with their SWS often experience cognitive impairments, including memory issues, and diminished sleep quality [4]. As such, researchers have also observed decreased SWS in the elderly and in patients with dementia [5]. The symptoms of inadequate SWS result in part because of less activity of the processes that typically occur during SWS, including glucose metabolism and energy restoration, hormone regulation, supporting immune response, and memory consolidation, all of which are important for our health and daily functioning [4]. Memory consolidation during SWS has recently become a larger area of sleep research as scientists examine how it occurs during SWS, gain new insights on slow wave mechanisms, and explore methods to improve SWS and sleep quality overall.
Memory Consolidation
People are naturally curious, though the pressure to learn successfully can be greater for students preparing for exams. There comes a point in every student’s life where they feel unprepared for a test and make the decision to do some late-night studying, hoping that they can improve their memory on a subject at the cost of their own sleep. In reality, losing sleep can make it harder to learn [3].
Memories are strengthened through a process called memory consolidation, which works by transferring new information from short-term memory to long-term memory [6]. Without memory consolidation, people could be at risk of forgetting subjects learned throughout the day. Memory consolidation is commonly believed to occur in two stages. In the two-stage model, the first stage occurs while a person is awake and the second stage occurs while they are asleep. During waking hours, all new information is taken in and recorded for short-term memory storage in the hippocampus, a subcortical brain structure important for memory. Simultaneously, at a much slower rate, the awake brain also begins to record the information in the cortex, where long-term memory storage occurs. When a person goes to sleep, the second stage begins, and memories are randomly replayed so that they can be stored as long-term memory in the cortex rather than as short-term memory in the hippocampus [6].
Think back to the beach and how waves become larger and less frequent the further out someone goes, similar to brain waves as we sink into deeper stages of sleep. SWS is characterized by waves similar to when we were further away from the beach’s shore [4]. The largest waves are slow oscillations, which have a frequency of less than one cycle per second, followed by slightly faster waves known as k-complexes and delta waves. Also present during SWS are spindles, which are small waves with high frequency, 10 to 15 cycles per second [4]. If the brain does not get the chance to experience SWS, information learned cannot be adequately reinforced and there is an increased risk of forgetting the new material. The slow oscillations that occur during SWS orchestrate the whole consolidation process. Slow oscillations are some of the largest, least frequent waves [6]. Their peaks are referred to as “up-states” and correspond to increased neuron firing, while their lowest points, called “down-states,” indicate neuron inactivity [6].
Researchers often use a method called targeted memory reactivation (TMR) to influence which memories are strengthened during the slow oscillations in SWS [6]. TMR works by pairing an auditory or olfactory cue with something the researchers want participants to learn [6]. Researchers then expose participants to those cues while they sleep to influence which memories are reactivated and improve their consolidation. One study had participants learn the locations of different objects in a picture, with each object paired with a related sound (for example, a picture of a cat would be paired with a meowing sound). Before going to sleep, all participants were tested on the locations of the objects. This pre-nap memory test served as a baseline measure. After this, all participants were hooked up to electroencephalograms (EEGs) and went to sleep. EEGs measure brain waves using sensors applied to your scalp to record the electrical activity of neurons in the brain. Once SWS was measured, half of the paired sound cues were played along with sounds that were not given during learning. Another memory test was given 10 minutes after the nap [6].
Using this method, the researchers were able to determine when memory consolidation occurs during slow oscillations [6]. When comparing the before and after-nap test scores, they found that participants had a better memory of objects’ locations whose paired sounds were played during SWS than those that were not [6]. Additionally, they found that the memory of participants improved the most when paired cues were played during the up-states of slow oscillations, indicating that memory consolidation occurs mostly during the up-states of SWS. They also found evidence suggesting that the time when the auditory cue is presented during slow oscillations is important for optimizing the effects of TMR. Researchers hypothesized that when the cues were played during the first half of down-states, the brain had time to process the sound so that when the up-states occurred, the brain could focus primarily on the reactivation and strengthening of the associated memory. This suggests that the cortex may be actively controlling the hippocampus in what is called a “feedforward interaction.” In this feedforward interaction, during up-states, the cortical neurons signal first, telling the hippocampus which memories to reactivate for consolidation. TMR may work in a similar fashion for influencing which memories are reactivated in the hippocampus [6].
All of this occurs during the second stage of memory consolidation while asleep, and as evidenced by the study, slow oscillations play a crucial role in the communication between the cortex and the hippocampus [6]. Memory reactivation during SWS occurs when slow oscillations trigger spindles between the thalamus and the cortex along with sharp-wave ripples in the hippocampus [6]. The sharp-wave ripples during SWS are another type of brain wave that occur between 150 to 250 cycles per second [7]. Looking at the ocean, these waves are very similar to ripples in the water, as they are small and fast. Research suggests these ripples indicate the reactivation of specific memories, since their patterns during SWS are the same as when the memory was initially recorded while awake [4]. The sharp-wave memory reactivation allows information to be further strengthened in the cortex as long-term memory. This communication is possible because the slow oscillations are highly synchronized, indicating neurons activate and inactivate as one [6]. Without this synchrony, different areas of the brain would not be able to communicate with each other during up-states as efficiently, and consolidation would not occur as well as it should.
Synchronizing the Brain
Attempting to determine the mechanisms behind the highly synchronized nature of slow wave activity, scientists turned to the claustrum, which is a thin structure located beneath the cortex with inputs from and outputs to nearly all areas of the cortex [8]. For many years, scientists have been attempting to study the function of the claustrum, which they hypothesize plays a role in consciousness, multisensory integration, and attention. Recently, it has been found that it may be involved in orchestrating the feedforward interaction between the cortex and hippocampus as memories are being transferred to long-term storage. Researchers have only just begun to explore the claustrum’s role in slow wave activity, but findings suggest that it initiates the down-state synchronously across the entire cortex, resulting in the neurons reactivating at the same time, creating the up-state of slow wave activity [8].
In the past, it has been difficult to study the claustrum due to its small size, but a Japanese research group led by Dr. Kimiya Narikiyo and colleagues at the RIKEN Brain Science Institute were recently able to create a special line of genetically engineered mice that make the claustrum easier to manipulate and research [8]. The claustrum typically contains both inhibitory and excitatory neurons. Inhibitory neurons send signals that make it more difficult for other neurons to generate action potentials and send their own signals, while excitatory neurons do the opposite and make it easier for other neurons to send out their own signals. The genetically modified mice allowed the researchers to focus on the excitatory claustral neurons [8].
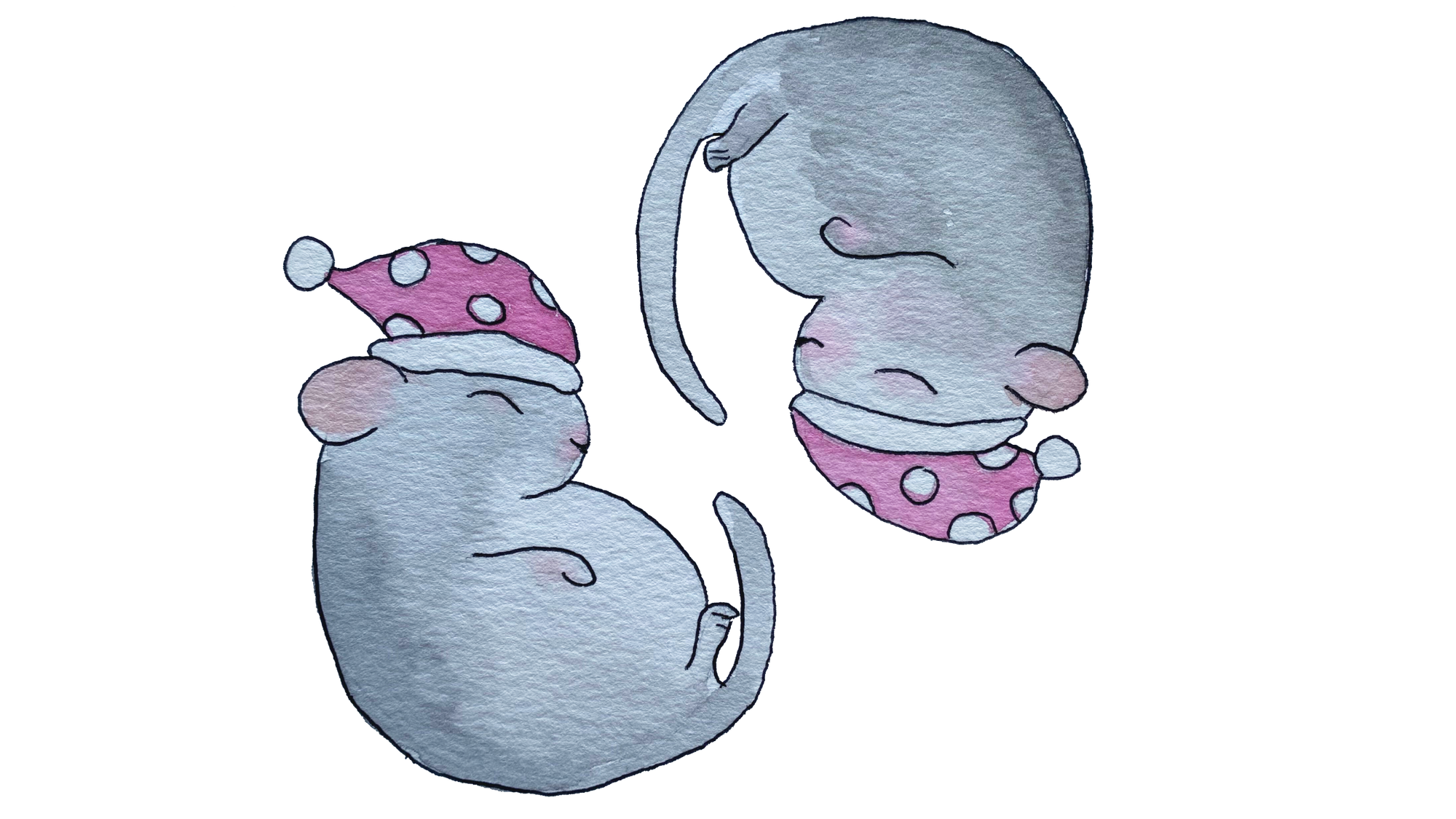
The group performed multiple experiments in order to learn more about the physiology of the claustrum, specifically which neurons sent signals to the excitatory claustral neurons and which ones received signals from them [8]. They found that the excitatory neurons in the claustrum received signals from multiple cortical areas all over the brain and a few subcortical areas, including the substantia innominata, the raphe nuclei, and parts of the thalamus. These structures play important roles in SWS and could potentially influence the claustrum’s activity during this sleep phase [8]. The thalamus is involved in memory consolidation during sleep, as it generates spindles between itself and the cortex during slow oscillations [6]. Additionally, the raphe nuclei, which contains a large network of inhibitory neurons, may play a role in generating SWS [9]. The substantia innominata, on the other hand, could have an opposite effect on the claustrum, as it plays a role in the desynchronization of brain waves and more active behaviors [10]. While these structures sent information to the claustrum, very few subcortical structures received input from the claustrum. The claustral excitatory neurons send signals reaching throughout the whole cortex [8]. While the claustrum does receive input from brain structures not involved in SWS, hinting at the other functions the it may perform, the fact that its excitatory neurons receive input from these structures involved in SWS may suggest that this small brain structure is regulated by these areas in order to begin or cease generation of slow wave activity [8].
After determining the neural connections to and from the claustral excitatory neurons, Narikiyo and their colleagues performed optogenetic stimulation of the mice brains in vitro and in vivo [8]. Optogenetic stimulation involves the use of high intensity light to trigger action potentials or signal firing in neurons. For in vitro experiments, the group used mouse brain slices of the claustrum and the insular cortex, a structure that lies deep within the cortex and plays a role in emotional integration and cognition [8,12]. By conducting these experiments, the research team hoped to determine the types of neurons affected by the claustrum. When they optogenetically stimulated the claustrum, the neurons in the structure sent signals to almost all other neurons in the insular cortex. However, out of all the neurons that received signals from the claustrum, the inhibitory neurons were primarily able to send out signals of their own [8]. This suggests that the claustrum’s involvement in consciousness and sleep is to “turn off” or silence large portions of the brain, creating the down-state across the entire cortex.
While conducting in vivo experiments, Narikiyo and colleagues looked at the effects of claustral optogenetic stimulation on the frontal cortex regions which had previously received significant amounts of input from the claustrum [8]. They found that when the excitatory claustral neurons were stimulated during SWS and quiet wakefulness, which is a more relaxed state that can be experienced lying awake with eyes closed, they caused a synchronized silencing of neuron firing in almost all areas in the frontal cortex, resembling a naturally occurring down-state of slow wave activity. Consistent with the in vitro brain slice experiments, optogenetic stimulation of claustral neurons resulted in the activation of primarily inhibitory neurons. The in vivo experiments revealed that immediately after these inhibitory neurons sent off a signal, almost all of the measured groups were suppressed. After a period of silence, these neurons reactivated at the same time, suggesting that the claustrum controls the up-state’s synchronous firing of cortical neurons by creating the slow wave down-state [8]. This discovery ultimately provides insight into how slow oscillations occur and become highly synchronized, allowing for better strengthening of neural connections and more efficient memory consolidation.
Improving Slow Wave Sleep
The more we know about the mechanisms behind SWS, the more we can look into ways we can improve it. We can do this by targeting different areas involved in the generation of slow wave activity and other characteristics of SWS. If we can improve our slow wave sleep, we can potentially improve our quality of sleep and even our memory consolidation. Beyond the scope of just the claustrum, there is current research looking at strategies to improve slow wave sleep. Some methods can only be used in lab settings, but there are plenty of others that you can try yourself.
One method used in sleep labs to induce slow wave activity is slow-oscillatory transcranial direct current stimulation (so-tDCS) [12]. This procedure uses electrodes placed on the brain to send electrical currents that match the patterns of slow wave activity during sleep with the intention of increasing the power and duration of SWS [12]. Studies have found that applying so-tDCS during sleep improves memory in adults [13]. The specific type of memory improved by this method is declarative memory, which is information related to facts and events. While stimulating slow oscillations in this manner is an invasive technique that cannot be done at home, it does have potential for treating memory decline in the elderly [13].
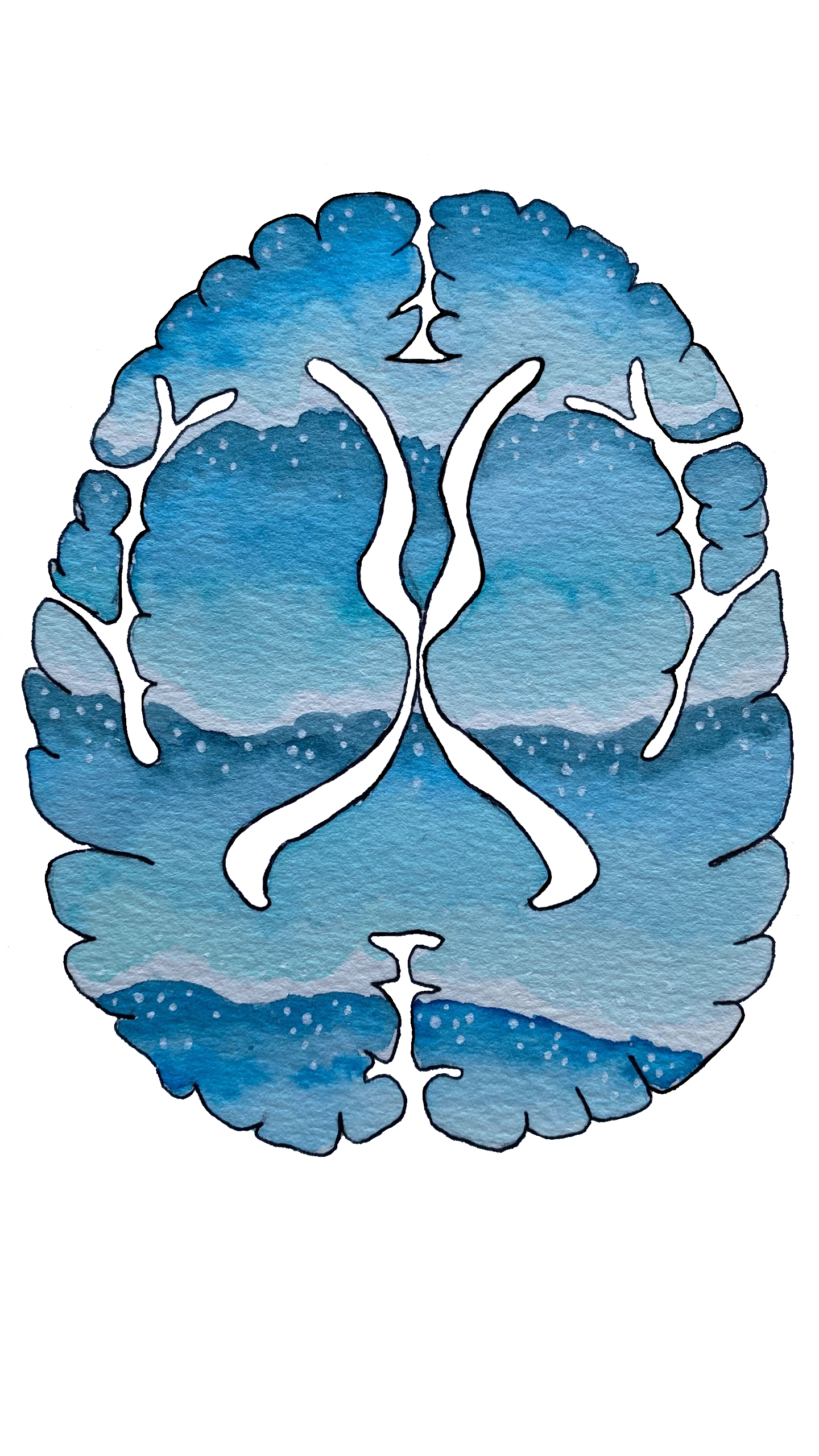
Auditory stimulation is another method that has been found to enhance SWS. One study played 50 millisecond bursts of pink noise during the up-phases of slow oscillations [14]. Pink noise contains all of the frequencies humans can hear, but the intensity of the sounds decrease as frequency increases [15]. When the pink noise was successfully timed to be in phase with the up-states, there was an increase in the number of slow oscillation cycles and amplitude of slow oscillations [14]. Additionally, people in the study who experienced auditory stimulation in their sleep after learning a task had better retention of word-pairs learned before sleeping. This improved memory was found to be associated with the percent of SWS experienced during sleep. However, when the pink noise auditory stimulation occurred during the slow oscillation down-states, slow oscillation activity was disrupted and did not improve memory consolidation [14]. Since this form of auditory stimulation requires knowing what phase of slow oscillation is occurring, it is hard to do it at home with success. However, scientists have been looking into forms of TMR that can be performed individually in the household [14].
Targeted memory reactivation shows the most benefits to memory when auditory stimulation is played before the up-states [6]. However, that does not mean auditory stimulation has no effects when cues are administered during other parts of slow oscillations [6]. One study attempted to see if a simple at-home TMR setup could improve the memory of participants over the course of three nights [16]. Native German-speaking participants were taught Dutch-German word pairs and then for the next three nights played an mp3 audio file that, after 30 minutes of silence, repeatedly played half of the Dutch words. This method was found to only significantly improve memory of words that were played during sleep for those who did not experience any sleep disturbances or awakenings at night. Further studies are required to better understand the relationship between TMR and sleep quality. The participants’ sleep quality may have been affected by study conditions such as wearing earphones at night [16]. Patience is important if you are looking for any benefits to unsupervised TMR because you will need to allow your brain to adjust to the new sleeping conditions and return to your baseline sleep quality.
Another method that has shown to have potential is hypnotism. Studies have found that listening to hypnotic suggestions while asleep to “sleep deeper” can help improve SWS [4]. Listening to hypnosis tapes about sleeping deeply right before or while sleeping almost doubles SWS and increases slow wave activity [17]. Women who experienced increased SWS due to hypnotic suggestion also reported improved sleep quality [17]. These benefits occur both in young adults aged 18-35 and in elderly women ages 60 and up [18]. Additionally, memory consolidation also improved in the elderly women who experienced hypnotic suggestions and may have the potential to improve cognition in the elderly [18]. Unfortunately, these results have only been observed in women who are highly suggestible to hypnosis and do not translate to women who are not. Furthermore, the effects of hypnosis on men’s SWS have yet to be studied [18]. Luckily, more recent research looking at both men and women has found that listening to “relaxing words” while sleeping is enough to improve SWS and sleep quality [19]. These relaxing words are not hypnotic suggestions, but are instead calming and reassuring words spoken in a soft, slow, and calm voice. The study began playing these words during the second sleep cycle, and they found that participants experienced deeper sleep and increased slow wave activity. They also reported better sleep quality and increased alertness after sleep [19].
Beyond using auditory stimulation during sleep, there are some lifestyle changes that have also shown to improve SWS. For example, reducing caffeine intake before bed is key for a good night’s rest. When caffeine is consumed, the caffeine binds to receptors in the brain meant for the neurotransmitter adenosine, blocking adenosine from performing its normal functioning in regulating sleep [20]. A study looking at the effects of taking 400 mg of caffeine before bed found that it decreased SWS [21]. This is because adenosine is able to bind to its receptors in the brain when caffeine is not present, and the longer you are awake, the more adenosine binds to receptors, resulting in an increase in slow waves experienced during SWS [20]. The study found that even consuming caffeine six hours before going to bed was enough to reduce sleep by over an hour [21]. Disturbances affecting sleep occur more as well, but they are only noticeably affected by those sleeping when taking caffeine three hours before and right before bedtime. As painful as it may sound, especially for students trying to get in some nighttime studying, caffeine consumption should be avoided in the hours prior to sleep [21].
More recently, scientists have also been looking into the effects of exercise on SWS and sleep quality. One study had a group of healthy, untrained men perform one hour of vigorous exercise before dinner [22]. They found that while self-reported sleep quality decreased in the “refreshness” category, participants’ SWS was improved. It is possible that participants did not notice an improvement in sleep quality because they were not used to the high intensity of the workout and the muscle soreness that resulted. However, past studies using moderate exercise have found improvements in subjective sleep quality [22]. While the duration of SWS decreased in the first sleep cycle, scientists found an increase in wave density and stability during SWS indicating an increase in sleep efficiency. The increased wave power suggests that the need for sleep was reduced faster in the early sleep phases compared to those who did not exercise before dinner [22]. So, for those looking to add exercise to their routine, moderation may be the key.
There are many strategies for improving SWS, memory, and sleep quality. Some of them are difficult to do outside of a lab setting, but more research is being done to increase the accessibility and generalizability of these methods. With all of the new information, it is up to the individual to find what strategy works best for them.
Further Implications
Scientists know where the ocean’s waves come from. They understand the importance of the moon in the changing of tides and how various energy sources contribute to the generation of waves at the beach. On the other hand, while it is understood what various brain waves signify, scientists have yet to fully understand sleep. Despite the emerging information on SWS and memory consolidation, there are still questions that remain largely unanswered. What signals the claustrum to initiate synchronized slow wave activity? How do the processes of memory consolidation in SWS versus REM interact? How do these methods of improving SWS affect different types of memory? As technology advances, these are some of the questions that scientists can begin to answer. Future research can look more into the claustrum’s role in neuron synchronization and how this may influence memory consolidation. Being able to safely explore these mechanisms involving the claustrum in humans would also be an important direction to take. This would aid researchers in understanding improvements for SWS and possible treatments for sleep disorders. Several of the sleep disorders that decrease SWS impact memory and sleep quality, though many causes behind these disorders remain a mystery. This is just one reason why research on the mechanisms of SWS is important. It could help uncover new ways to help treat various disorders and also slow cognitive decline in the elderly. Slow-oscillatory tDCS already shows promise for treating cognitive decline, however further exploration of its benefits needs to occur along with increasing the accessibility of this method as a treatment.
Sleep is a universal activity that everybody needs to do to survive. That is why it is important to do what you can to get the most out of your sleep, be it through meditative sleep playlists or by making lifestyle changes that will improve the quality of your slow wave sleep. As we grow, so does our knowledge, and now that we understand slow wave sleep is more important for our memory than perhaps originally believed, we can move even closer to unlocking the many mysteries of sleep.
References
- Moore H, Lega BC, Konopka G. Riding brain "waves" to identify human memory genes. Curr Opin Cell Biol. 2022 Oct;78:102118. doi: 10.1016/j.ceb.2022.102118. Epub 2022 Aug 7. PMID: 35947942.
- Patel, A.K., Reddy, V., Shumway, K.R., & Araujo, J.F. (2022). Physiology, Sleep Stages. StatPearls [Internet]. https://www.ncbi.nlm.nih.gov/books/NBK526132/
- Sleep Deprivation and Deficiency: How Sleep Affects Your Health. (2022). NHLBI. https://www.nhlbi.nih.gov/health/sleep-deprivation/health-effects
- Léger, D., Debellemaniere E., Rabat A., Bayon V., Benchenane K., & Chennaoui M. (2018). Slow-wave sleep: From the cell to the clinic. Sleep Medicine Reviews, 41(1), 113-132. https://doi.org/10.1016/j.smrv.2018.01.008
- Enrica, B., Di Coscio, E., Meastri, M., Carnicelli, L., Tsekou, H., Tiberio Economou, N., Paparrigopoulos, T., Bonakis, A., Papageorgiou, S.G., Vassilopoulos, D., Soldatos, C.R., Murri, L., & Ketones, P.Y. (2012). Differences is EEG Delta Frequency Characteristics and Patterns in Slow-Wave Sleep Between Dementia Patients and Controls. Journal of Clinical Neurophysiology, 29(1), 50-54. https://doi.org/10.1097/WNP.0b013e318246b56d
- Batterink, L.J., Creery, J.D., & Paller, K.A. (2016). Phase of Spontaneous Slow Slow Oscillations during Sleep Influences Memory-Related Processing of Auditory Cues. JNeurosci, 36(4), 1401-1409. https://doi.org/10.1523/JNEUROSCI.3175-15.2016
- Csicsvari J & Dupret D. (2014). Sharp wave/ripple network oscillations and learning-associated hippocampal maps. Philos Trans R Soc Lond B Biol Sci, 369(1635). https://doi.org/10.1098/rstb.2012.0528
- Narikiyo, K., Mizuguchi, R., Ajima A., Shiozaki M., Hamanaka H., Johansen J. P., Mori K., & Yoshihara Y. (2020). The claustrum coordinates cortical slow-wave activity. Nature Neuroscience, 23, 741-753. https://doi.org/10.1038/s41593-020-0625-7
- Raphe Nuclei. (n.d.). ScienceDirect. https://www.sciencedirect.com/topics/neuroscience/raphe-nuclei
- Substantia innominata. (n.d.). ScienceDirect. https://www.sciencedirect.com/topics/medicine-and-dentistry/substantia-innominata
- Kortz, M. W. & Lillehei, K. O. (2021). Insular Cortex. National Library of Medicine. https://www.ncbi.nlm.nih.gov/books/NBK570606/
- Zhang, Y. & Gruber, R. (2019). Can Slow-Wave Sleep Enhancement Improve Memory? A Review of Current Approaches and Cognitive Outcomes. YJBM, 92(1), 63-80. PMID: 30923474
- Westerberg, C.E., Florczak, S.M., Weintraub, S., Mesulam, M.M., Marshall, L., Zee, P.C., & Paller, K.A. (2015) Memory improvement via slow-oscillatory stimulation during sleep in older adults. Neurobiology of Aging, 36(9), 2577-2586. https://doi.org/10.1016/j.neurobiolaging.2015.05.014
- Ngo, H. V., Martinetz, T., & Born, J. (2013). Auditory Closed-Loop Stimulation of the Sleep Slow Oscillation Enhances Memory. Neuron, 78(3), 545-553. https://doi.org/10.1016/j.neuron.2013.03.006
- Britannica, T. Editors of Encyclopaedia (2013, September 23). noise. Encyclopedia Britannica. https://www.britannica.com/science/noise-acoustics
- Göldi, M. & Rasch, B. (2019). Effects of targeted memory reactivation during sleep at home depend on sleep disturbances and habituation. npj Science of Learning, 4, 5. https://doi.org/10.1038/s41539-019-0044-2
- Cordi, M.J., Psych, D., Schlarb A.A., & Rasch, B. (2014). Deepening Sleep by Hypnotic Suggestion. Sleep, 37(6), 1143-1152. https://doi.org/10.5665/sleep.3778
- Cordi, M.J., Hirsiger, S., Mérillat, S., & Rasch, B. (2015). Improving sleep and cognition by hypnotic suggestion in the elderly. Neuropsychologia, 69, 176-182. https://doi.org/10.1016/j.neuropsychologia.2015.02.001
- Beck J., Loretz E., & Rasch B. (2021). Exposure to relaxing words during sleep promotes slow-wave sleep and subjective sleep quality. Sleep, 44(11). https://doi.org/10.1093/sleep/zsab148
- Clark I & Landolt HP. (2016). Coffee, caffeine, and sleep: A systematic review of epidemiological studies and randomized controlled trials. Sleep Medicine Reviews, 31, 70-78. https://doi.org/10.1016/j.smrv.2016.01.006
- Drake C., Roehrs T., Shambroom J., & Roth T. (2013). Caffeine Effects on Sleep Taken 0, 3, or 6 Hours before Going to Bed. Journal of Clinical Sleep Medicine, 9(11). https://doi.org/10.5664/jcsm.3170
- Park I., Díaz J., Matsumoto S., Iwayama K., Nabekura Y., Ogata H., Kayaba M., Aoyagi A., Yajima K., Satoh M., Tokuyama K., & Vogt K.E. (2021). Exercise improves the quality of slow-wave sleep by increasing slow-wave stability. Scientific Reports, 11(1), 4410. https://doi.org/10.1038/s41598-021-83817-6