Over a century ago, Paul Ehrlich injected blue dye into the bloodstream of mice to view their biological structures. After dissecting the mice, he was surprised to find that all the tissues in their bodies were stained, except the brain [1]. This phenomenon was soon explained by the blood brain barrier (BBB), which consists of tightly packed cells lining the blood vessels that run through the brain. This selectively permeable membrane prevents microbes, toxins, and other large molecules flowing through the bloodstream from entering the brain and disturbing its natural state. The BBB is an evolutionary response to the brain’s hypersensitive properties–any small pathogen introduced to the brain can have extremely adverse effects on its function and health.
Creating artificial molecules that can cross the BBB is one of the foremost challenges in neuroscience. Many drug delivery systems designed to treat brain pathologies like Alzheimer’s disease, Parkinson’s disease, and brain cancer are dependent on being able to penetrate this barrier to reach the affected areas of the brain [2]. Recently, a team of researchers led by post-doctoral fellow Adrian Joseph from University College London discovered a mechanism that would improve BBB permeation, which may have far-reaching effects in medicine. Their innovation entailed adding precisely engineered properties to small, self-driving molecules, often referred to as nanoswimmers, to make penetration possible.
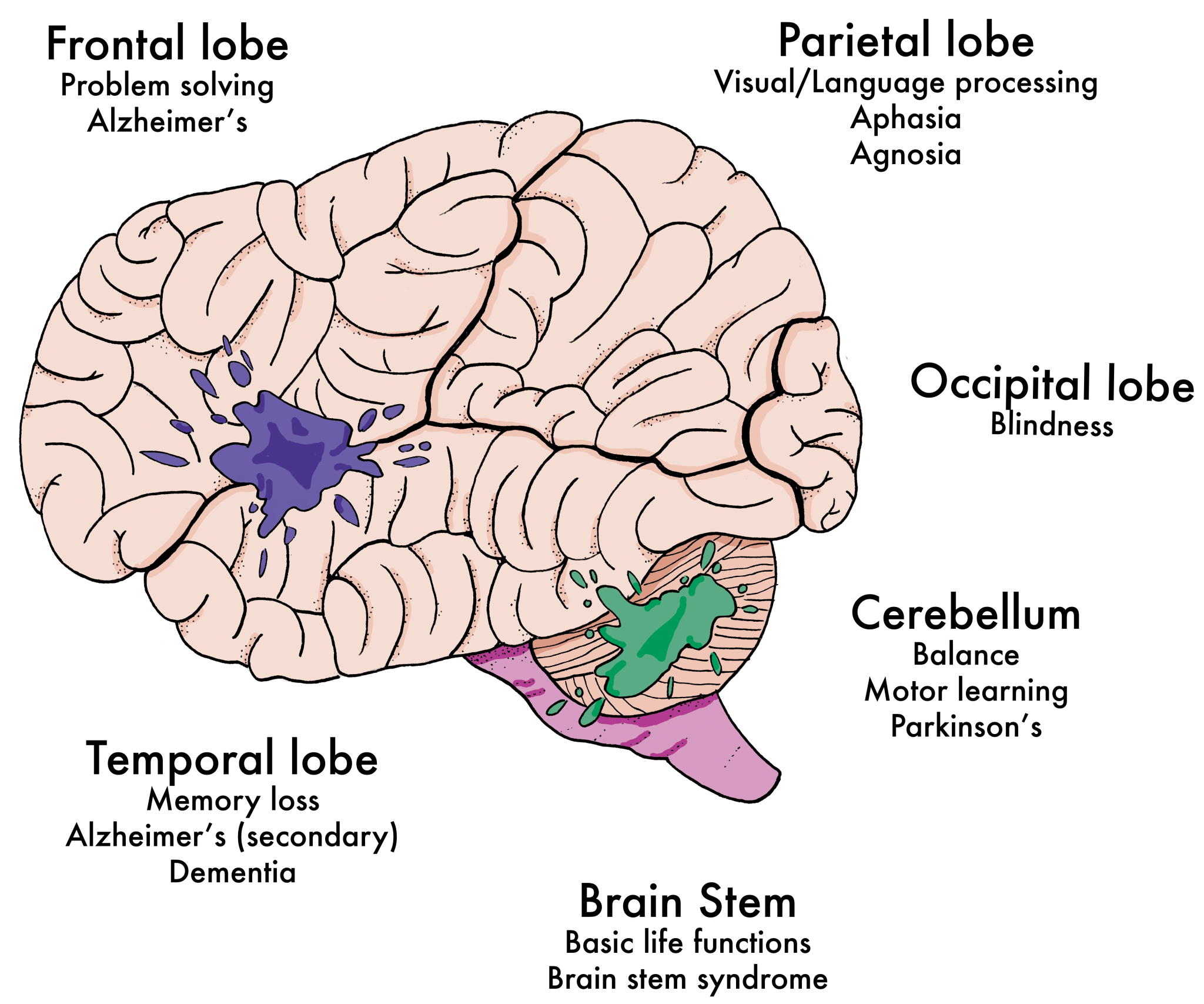
The Novel Nanoswimmers
The nanoswimmers developed by Joseph and colleagues fall under a group of molecules called “polymersomes”—tiny, artificial vesicles that encapsulate a substance [3]. Imagine a water balloon: water balloons are good at containing water because the rubbery balloon surrounding the water is not easy to rupture and creates an obstacle for outside particles. Similarly, polymersomes have a physical membrane that separates the contained substance from other molecules and cells in the body. This membrane shields the nanoswimmers from external materials so that the encapsulated substance can be solely administered in the brain. Other types of polymersomes already have many applications in drug delivery to different parts of the body; however, only these nanoswimmers have been engineered to traverse the BBB [5].
The uniqueness of the nanoswimmers comes from the properties of their membranes. These artificial membranes are created from native cell components, and their motion is modeled after cells in the body, distinguishing them from foreign particles [4]. As a result, when nanoswimmers are injected into the bloodstream, they do not elicit a foreign body response, which avoids an immune system reaction. In addition, nanoswimmers have the ability to release drugs only after traversing the BBB by gauging the external environment through their membranes and identifying specific brain chemicals.
Movement Mechanisms
Perhaps the most distinct quality of the nanoswimmers is that they traverse the BBB through chemotaxis. Chemotaxis involves the movement of organisms, cells, or molecules in response to the chemicals in their immediate environment and is a prevalent strategy in natural systems, used by sperm, white blood cells, and various types of bacteria [3]. A common example of chemotaxis is the attraction of fruit flies toward fruit. Fruit flies are able to sense and migrate in the direction of ripened fruit through their strong olfactory sense. Think of the body’s chemical environment as containing fruit with different levels of ripeness. The nanoswimmer is like a fruit fly navigating towards the scent of the ripest fruit scent it can detect. However, instead of responding to a ripened fruit, nanoswimmers move in response to glucose, the most prominent source of energy in the brain.
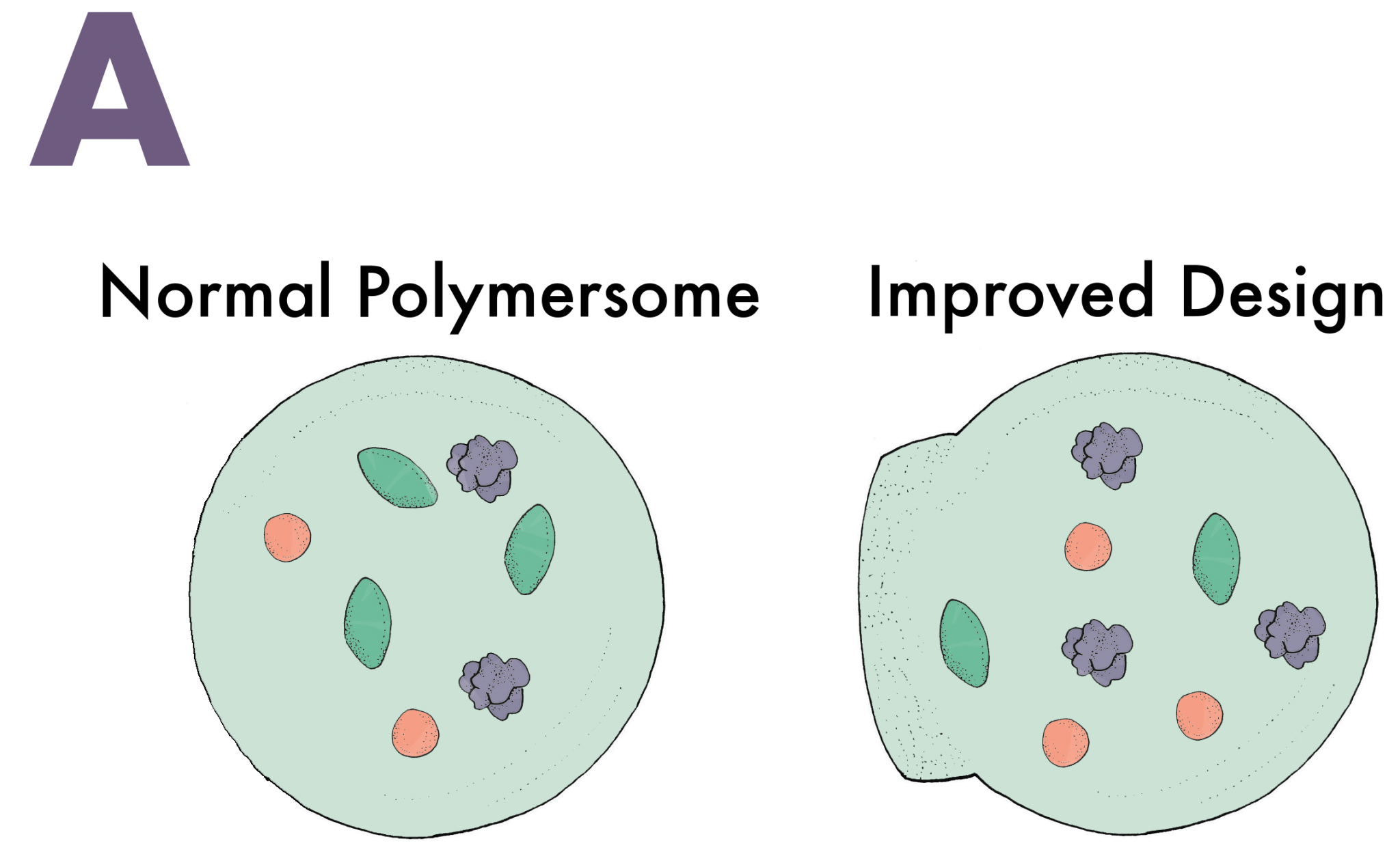
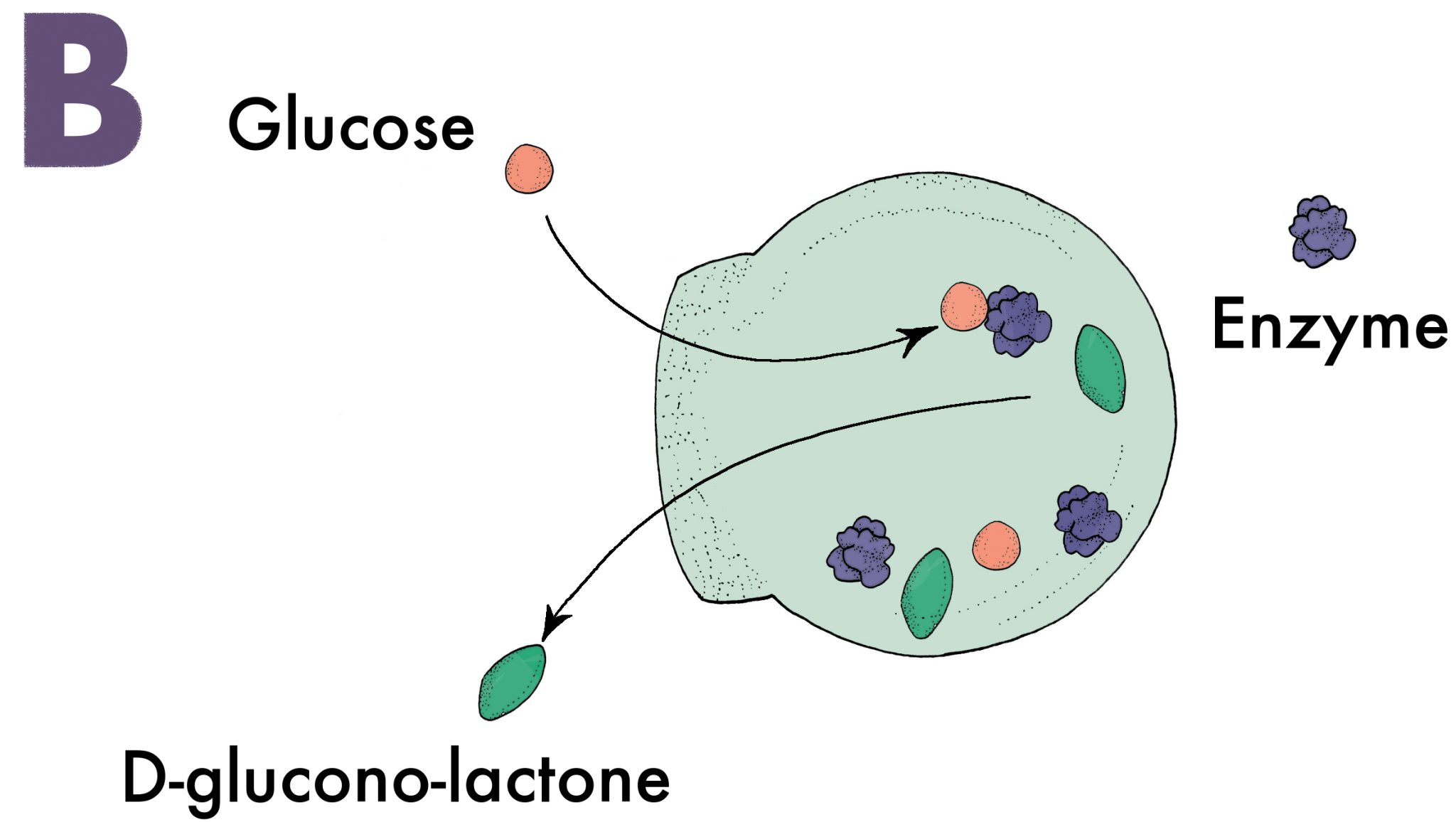
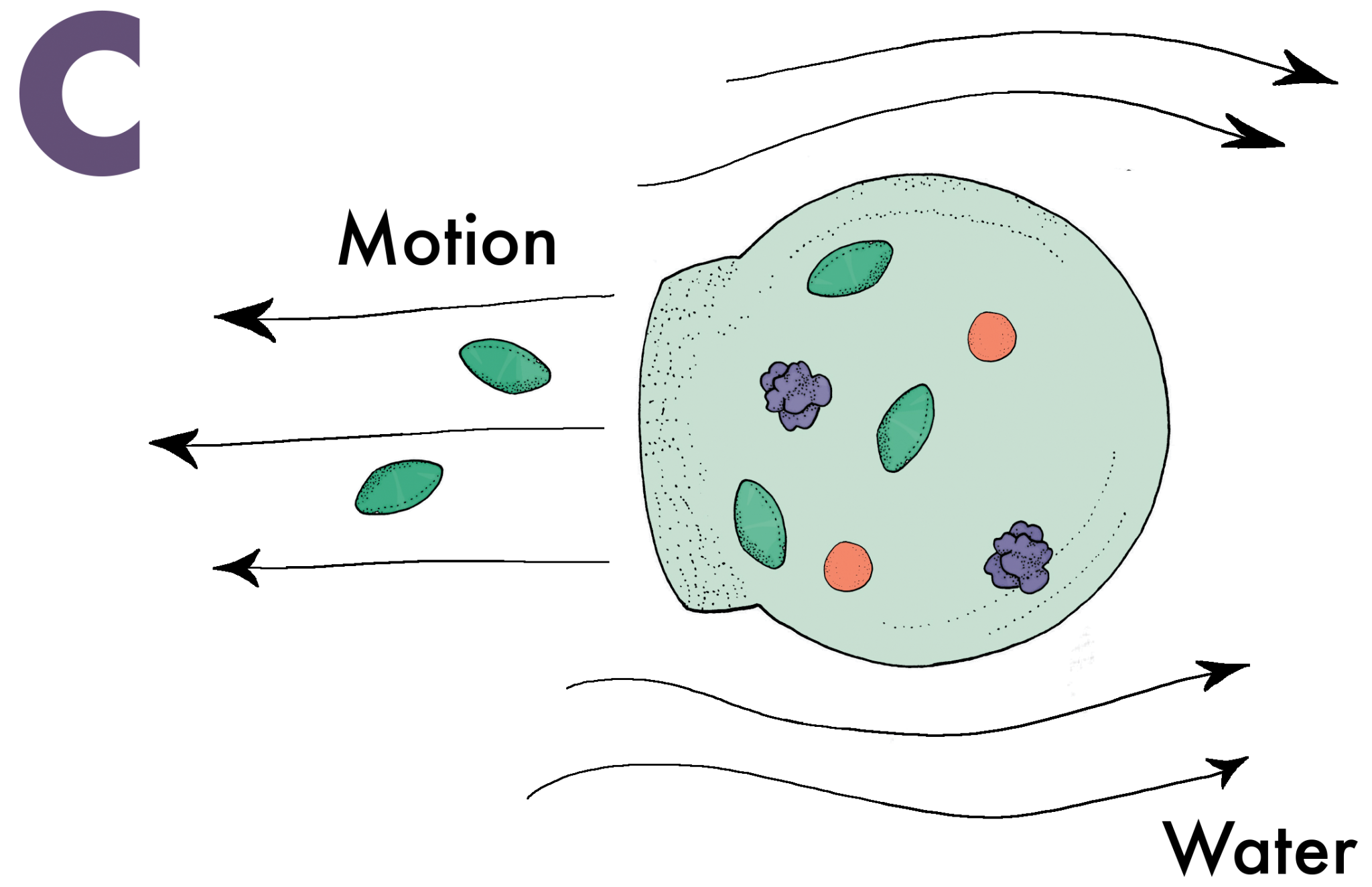
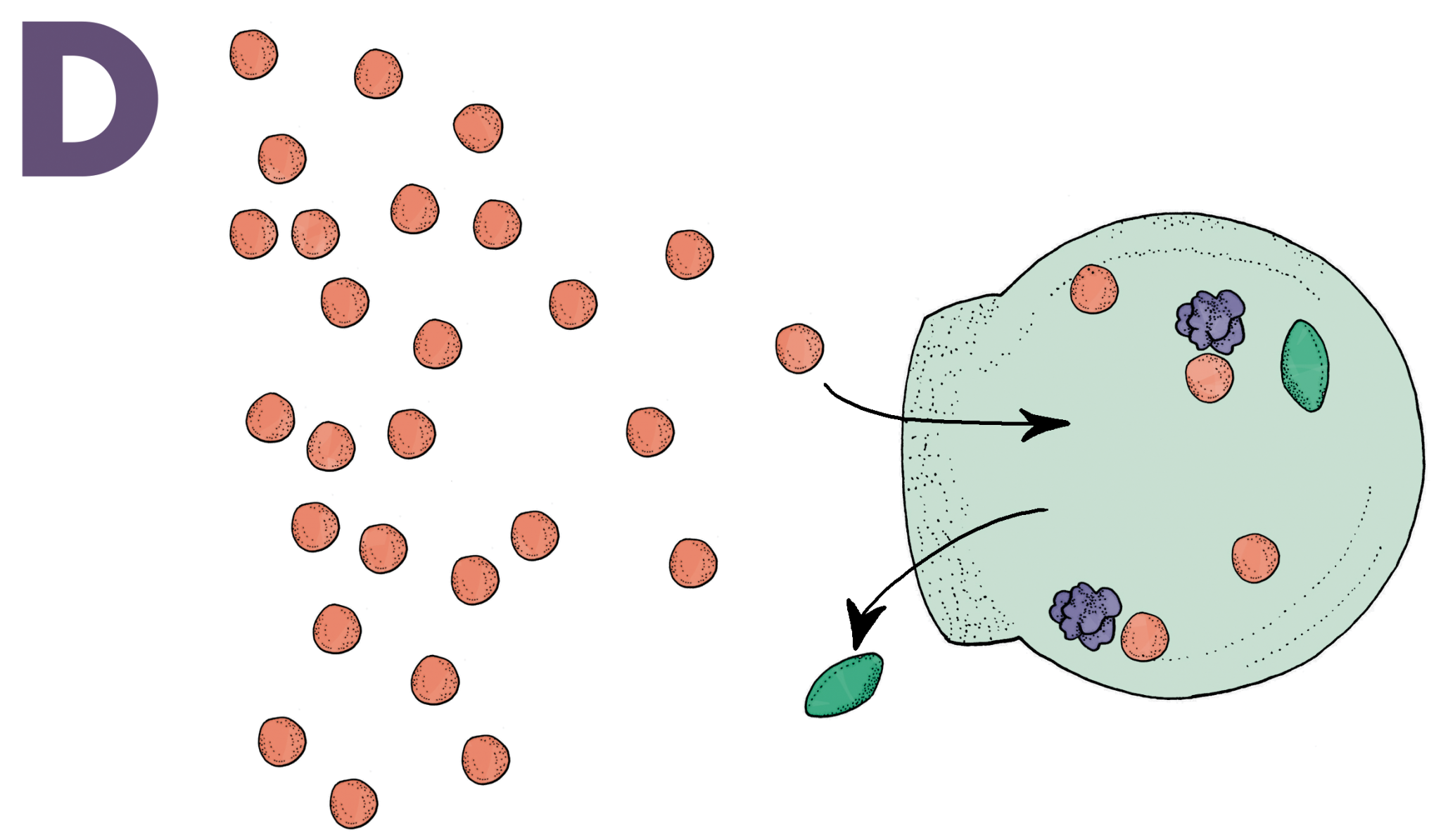
Seventy percent of the glucose humans consume is burned by the brain. Unsurprisingly, it is one of the few molecules capable of penetrating through the BBB. Nanoswimmers take advantage of this and slide their way through the BBB with glucose [6]. A vesicle without any means of directing its movement would follow a random path through a solution. Nanoswimmers, however, are designed to have an asymmetric shape that is semipermeable in one area, a critical feature for creating directed motion (Image A). When glucose diffuses through the semipermeable layer of the vesicle, an enzyme inside the vesicle called glucose oxidase converts the glucose into D-glucono-lactone and hydrogen peroxide (Image B). Once the products are created, they exit through the semipermeable layer and displace the extracellular fluids they come into contact with. These fluids are sent to the other side of the vesicle, which propels the nanoswimmer in the direction of the semipermeable layer (Image C). Naturally, when there is an increased amount of glucose, indicating a glucose concentration gradient, the vesicle will go farther and faster in that direction (Image D). Most of the glucose in the blood vessels of the brain traverses through the BBB, and nanoswimmers are able to follow closely behind to ultimately administer the desired drugs in the brain.
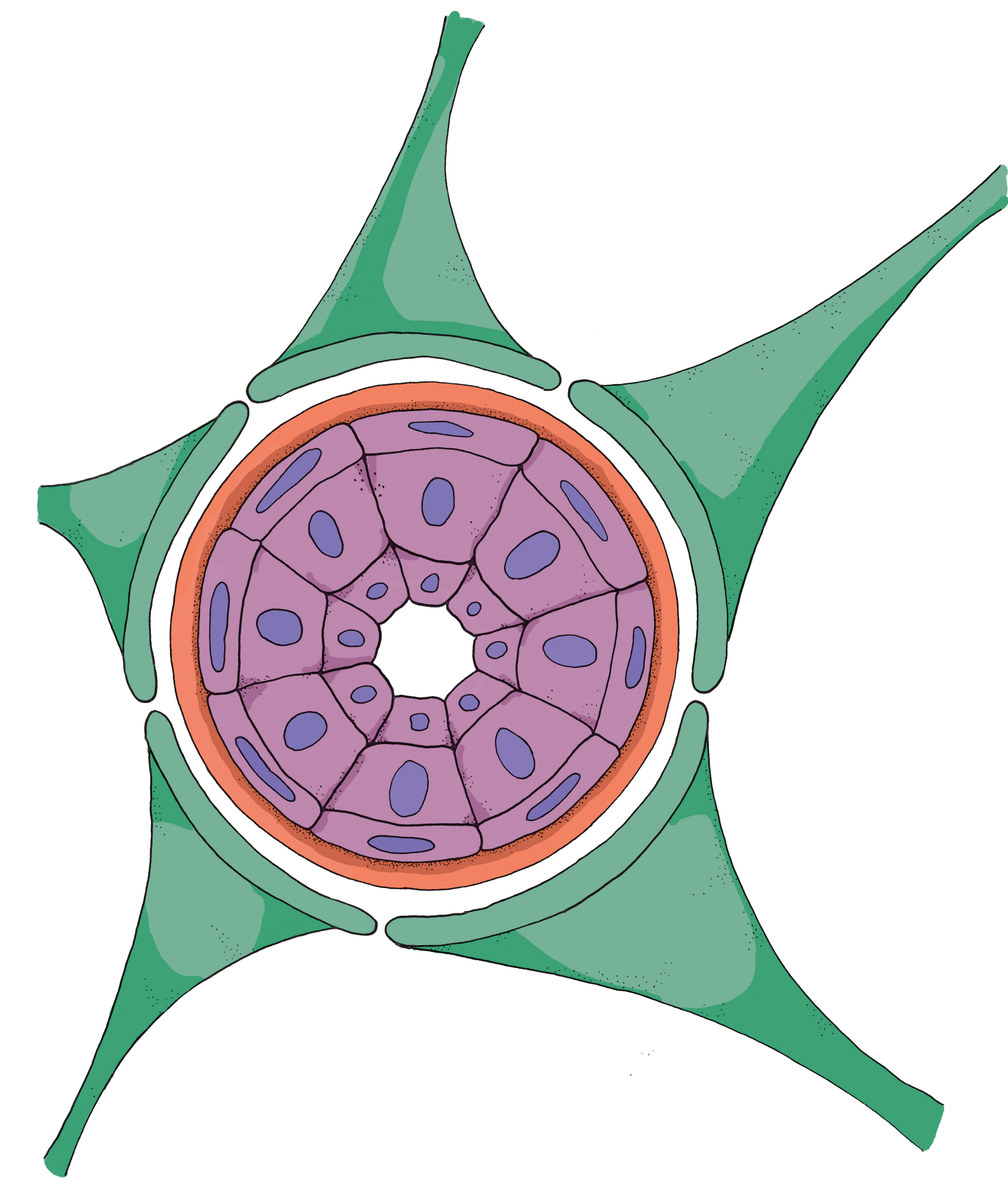
Looking Into the Future
Although this innovative technique of traversing the BBB is a breakthrough in the field, the system has many limitations that prevent it from being applied clinically. One limitation is that the nanoswimmer system works effectively until it traverses the BBB, but afterward, the administration of the substrate contained in the vesicle is very slow and unspecific. Research labs are working to develop a more effective nanoswimmer that can administer drugs in localized areas of the brain [7]. Neurological diseases like Parkinson’s and Alzheimer’s are significantly different and require drug administration to specific parts of the brain. In addition, there are many different types of brain cells, and releasing chemicals will affect these cells in different ways [2].
Other scientists have recognized the benefits of nanoswimmers and are attempting to utilize them in their research. University of Washington professor William Banks hopes to use this method for the delivery of anticancer drugs for brain tumors [7]. “They’ve used these natural mechanisms to make the vesicles [polymersomes] mobile, and the delivery rate of the vesicles into the brain is astounding,” Banks says. He adds that they have created a “significant advance that is on the right path” [6]. With continued efforts, more scientists are hoping to move this idea into a clinical phase within a matter of years [8]. Many have recognized that this innovation could lead researchers to break through even more scientific barriers.
References
- Ballabh, P., Braun, A., & Nedergaard, M. (2004, June). The blood-brain barrier: an overview: structure, regulation, and clinical implications. Retrieved from https://www.ncbi.nlm.nih.gov/pubmed/
- Saraiva, C. (2016, May 18). Nanoparticle-mediated brain drug delivery: Overcoming blood–brain barrier to treat neurodegenerative diseases. Journal of Controlled Release. Retrieved from https://www.sciencedirect.com/science/article/pii/S0168365916303236
- Kamat, N. P., Katz, J. S., & Hammer, D. A. (2011, July 07). Engineering Polymersome Protocells. The Journal of Physical Chemistry Letters. Retrieved from https://www.ncbi.nlm.nih.gov/pmc/articles/PMC3220919/
- Lee, J. S., & Feijen, J. (2011, October 14). Polymersomes for drug delivery: Design, formation and characterization. Therapeutic Delivery. Retrieved from http://www.sciencedirect.com/science/article/pii/S0168365911009576
- Singh, R., & Lillard, J. W. (2009, June). Nanoparticle-based targeted drug delivery. Retrieved from https://www.ncbi.nlm.nih.gov/pmc/articles/PMC3249419/
- Costandi, M. (2017, August 07). Drug-Carrying "Nanoswimmers" Could Slither Past the Brain's Cellular Defenses. Scientific American. Retrieved from https://www.scientificamerican.com/article/drug-carrying-ldquo-nanoswimmers-rdquo-could-slither-past-the-brain-rsquo-s-cellular-defenses/
- Upadhyay, R. K. (2014, July 20). Drug Delivery Systems, CNS Protection, and the Blood Brain Barrier. BioMed Research International. Retrieved from https://www.ncbi.nlm.nih.gov/pmc/articles/PMC4127280/
- Joseph, A., Contini, C., Cecchin, D., Nyberg, S., Ruiz-Perez, L., Gaitzsch, J., Battaglia, G. (2017, August 01). Chemotactic synthetic vesicles: Design and applications in blood-brain barrier crossing. Biomedical Engineering. Retrieved December 06, 2017, from http://advances.sciencemag.org/content/3/8/e1700362.full