In the 1800s, neuroscientist Ramon y Cajal researched the cellular composition of the brain, leading to an idea known as the “neuron doctrine.” In essence, it is the idea that the neuron is the fundamental unit of the brain. However, while neurons certainly play an important and central role, they are not the only cells at work. Our nervous system is also home to non-neuronal cells, called glia. Unlike neurons, these glial cells can’t fire action potentials, which are the nervous system’s main mode of communication. Historically, neuroscientists thought glia, meaning “glue” in Greek, primarily provided structural support for neurons. Thus, traditional models of brain function didn’t include these “glue” cells. But research has challenged this assumption in the past years as more complex functions of glial cells emerge.
Astrocytes, a type of glial cell, are excellent examples of the variety in glial cell function. Astrocytes remove toxins, provide nutrients to neurons, and regulate blood flow [1]. It has also been suggested that astrocytes are associated with multiple neurological disorders, including migraines, ALS, and Parkinson’s disease [2]. More recently, astrocytes have been scrutinized for their possible involvement in epilepsy.
Epilepsy is a disorder characterized by chronic seizures, which are large, synchronized bursts of abnormal neural activity in the brain, due in part to over-excited neurons. The seizures may remain localized in particular regions of the brain, a category known as partial seizures, or they can spread from their origin, which characterizes generalized seizures. Depending on where they occur in the brain, they can have a broad range of effects, including motor spasms and impaired consciousness. The diversity in types and causes of epilepsy make the condition difficult to study. Many forms of epilepsy are highly resistant to treatment, with nearly a third of epileptic patients unable to manage their seizures with medication [3].
One reason for investigating how astrocytes may be involved in epilepsy lies in a rare disorder called Alexander’s disease. Symptoms of infantile Alexander’s disease include an abnormally large head, developmental impairments, and seizures [4]. On the molecular level, the disease involves overproduction of GFAP, an astrocytic protein, although the protein’s specific role in the disease is unknown [4]. The presence of astrocyte dysfunction, and the fact that those with Alexander’s disease experience seizures, suggests that astrocytes may be involved in other seizure disorders, such as epilepsy.
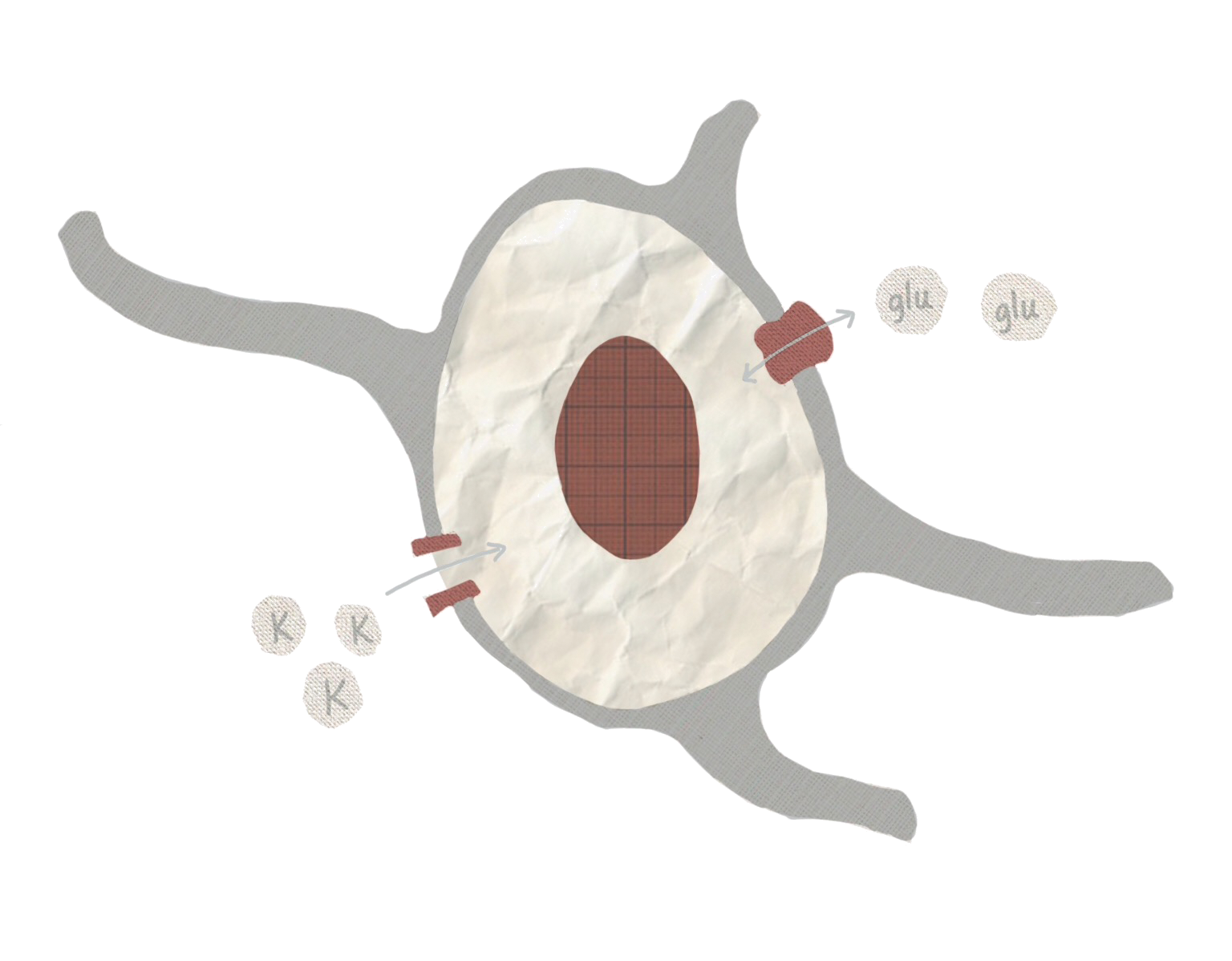
One important function that astrocytes preform, which may have a role in seizures, is removing excess potassium ions from outside cells [3]. Neurons rely on specific ratios of extracellular and intercellular potassium to regulate neural activity. When the concentration of potassium outside neurons is too high, neurons can become more likely to fire. This is particularly notable because over-excitable neurons are one characteristic of epilepsy, and too much extracellular potassium can cause seizures [5]. Astrocytes can maintain potassium levels by bringing potassium out of the extracellular space and into the astrocyte by using potassium transporter proteins on their cell membranes. One study, lead by Dr. Vinita and Alexander Thrane, demonstrated how ammonia, a seizure inducing substance, may disrupt this transporter [6]. Interrupting the function of the transporter could increase the amount of potassium outside of cells and may explain how ammonia causes seizures [6].
Dysfunction in maintaining potassium levels is not the only way in which astrocytes may contribute to epilepsy. Astrocytes are also be involved with the neurotransmitter glutamate. Typically, glutamate has an excitatory effect on neurons when released, so excess glutamate could lead to over-excited neurons. High levels of glutamate have been implicated in temporal lobe epilepsy, and glutamate release from astrocytes has been shown to excite surrounding neurons [7][8]. Neurotransmitters like glutamate are normally only released by neurons, but when astrocytes in cell cultures are exposed to calcium, they also release glutamate [9]. Interestingly, some epilepsy treatments actually inhibit calcium signaling in astrocytes [7]. Calcium likely serves an important role in neurotransmitter release, similar to a process that occurs in neurons, although the biological mechanism may differ somewhat in astrocytes [10]. Despite these findings, there is still ongoing debate over whether or not astrocytes can release glutamate in living organisms [11].
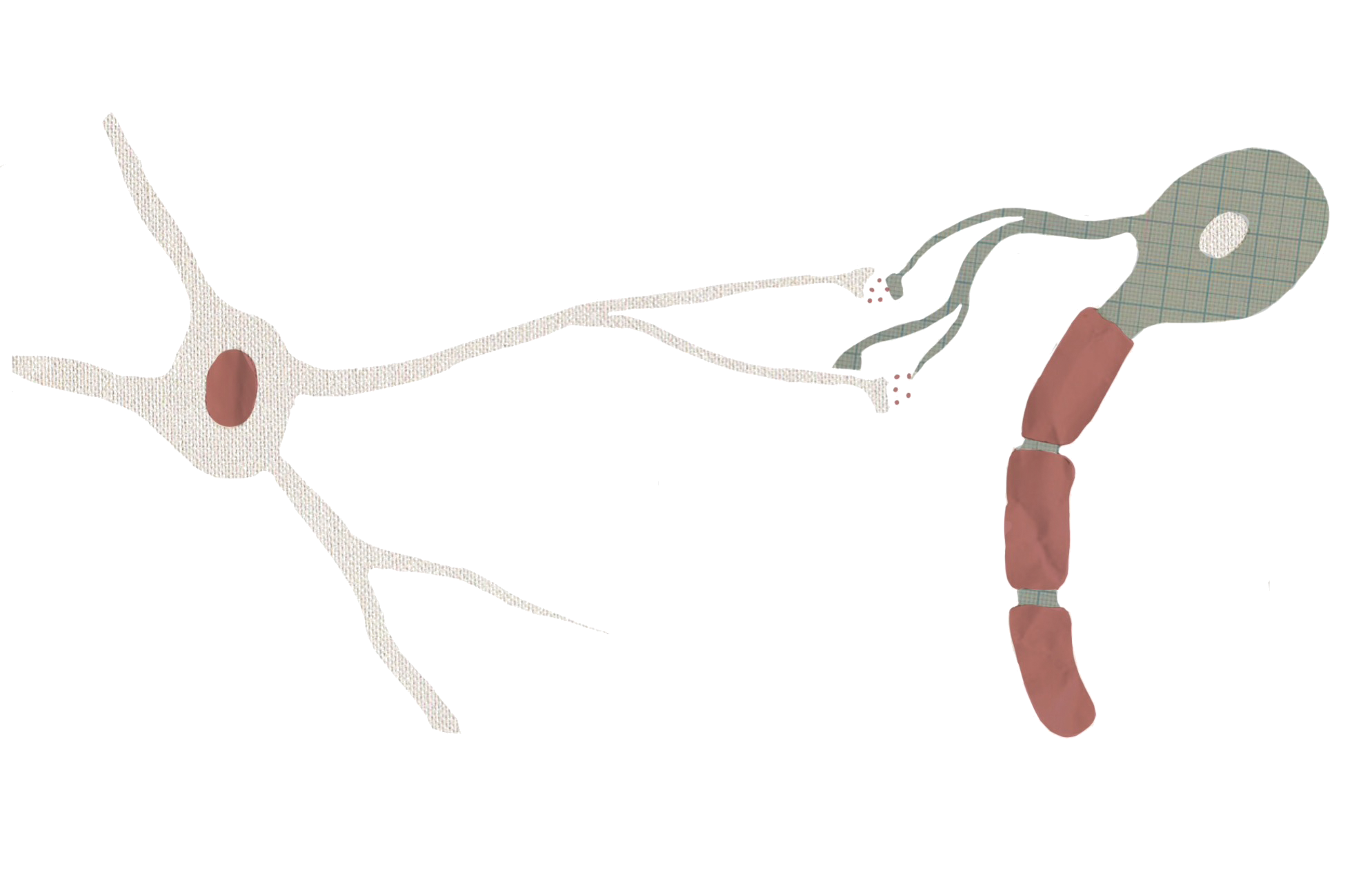
Astrocytes can also moderate glutamate levels by removing the neurotransmitter from the extracellular space and converting it to a different molecule using an enzyme called glutamine synthase [8]. Inadequate amounts of this enzyme can lead to less glutamate uptake by astrocytes. Glutamate that isn’t taken up and broken down could potentially interact with neurons and increase their excitability. One experiment showed that disabling glutamine synthase in animals can cause seizures [8]. Another study found that in mesial temporal lobe epilepsy, a type of epilepsy partially characterized by cell loss in the hippocampus, astrocytes had significantly lower levels of glutamine synthase in affected regions, as well as high levels of extracellular glutamate [12].
While research indicates that astrocytes might be more important than previously believed, they are not the only contributing factors. Epilepsy is a condition caused by a variety of diseases and injuries, and the scientific community is only beginning to understand its complexities. However, evidence increasingly suggests that the often-overlooked astrocytes have a role to play. While epilepsy is often described as a disease of highly excitable neurons, research shows the reality is much more complicated. Neurons are hardly the only cell of interest in the epileptic brain.
References
- Ransom, Behar, & Nedergaard. (2003). New roles for astrocytes (stars at last). Trends in Neurosciences, 26(10), 520-522.
- Sofroniew, M., & Vinters, V. (2010). Astrocytes: Biology and pathology. Acta Neuropathologica, 119(1), 7-35.
- Rao, & Lowenstein. (2015). Epilepsy. Current Biology, 25(17), R742-R746.
- Michael Brenner, Anne B. Johnson, Odile Boespflug-Tanguy, Diana Rodriguez, James E. Goldman, & Albee Messing. (2001). Mutations in GFAP, encoding glial fibrillary acidic protein, are associated with Alexander disease. Nature Genetics,27(1), 117.
- Seifert, & Steinhäuser. (2011). Neuron–astrocyte signaling and epilepsy. Experimental Neurology,Experimental Neurology.
- Vinita Rangroo Thrane, Alexander S Thrane, Fushun Wang, Maria L Cotrina, Nathan A Smith, Michael Chen, . . . Maiken Nedergaard. (2013). Ammonia triggers neuronal disinhibition and seizures by impairing astrocyte potassium buffering. Nature Medicine, 19(12), 1643.
- Guo-Feng Tian, Hooman Azmi, Takahiro Takano, Qiwu Xu, Weiguo Peng, Jane Lin, . . . Maiken Nedergaard. (2005). An astrocytic basis of epilepsy.Nature Medicine, 11(9), 973.
- Wetherington, Serrano, & Dingledine. (2008). Astrocytes in the Epileptic Brain. Neuron, 58(2), 168-178.
- Vladimir Parpura, Trent A. Basarsky, Fang Liu, Ksenija Jeftinija, Srdija Jeftinija, & Philip G. Haydon. (1994). Glutamate-mediated astrocyte–neuron signalling. Nature, 369(6483), 744.
- Malarkey, & Parpura. (2008). Mechanisms of glutamate release from astrocytes. Neurochemistry International, 52(1-2), 142-154.
- Nicola B. Hamilton, & David Attwell. (2010). Do astrocytes really exocytose neurotransmitters? Nature Reviews Neuroscience, 11(4), 227.
- Eid, Thomas, Spencer, Rundén-Pran, Lai, Malthankar, . . . De Lanerolle. (2004). Loss of glutamine synthetase in the human epileptogenic hippocampus: Possible mechanism for raised extracellular glutamate in mesial temporal lobe epilepsy. The Lancet,363(9402), 28-37.