Imagine a world where cherished memories vanish like whispers in the wind, leaving behind only fragments of a once vibrant existence. Alzheimer's disease, a relentless force that steals away our most treasured recollections, plunges individuals and their loved ones into an unyielding labyrinth of confusion and heartache. This insidious condition, characterized by the progressive degeneration of the brain, robs its victims of their identities, memories, and ultimately, their very essence. As we delve into the depths of this enigmatic disorder, we embark on a harrowing journey to unravel its mysteries, to understand the devastating impact it has on individuals and families, and to discover a glimmer of hope in the relentless pursuit of a cure.
Not much is known about the reason or nature of the onset of Alzheimer’s disease (AD). Recently, the disease has been associated with the misfolding of proteins, called amyloid beta plaques, and the deposition of proteins called phosphorylated tau starting in the hippocampus, which is a region of the brain associated with short term memory consolidation [1]. As a result, this age dependent neurodegenerative disorder causes short term memory loss and spatial memory problems. Right now, there is no cure for the disease. However, as Alzheimer’s disease research develops and expands, neurotransmitters have become a growing topic of conversation in relation to the onset and development of AD [2]. Neurotransmitters are chemicals that allow for communication between neurons and other cell types [3].
One neurotransmitter, acetylcholine, is necessary for short-term memory and learning in the central nervous system, which consists of the brain and spinal cord [2]. Depending on the neuronal pathway, acetylcholine has both inhibitory and excitatory responses – it is able to either stimulate or block a response. The inhibitory neurons play an important role in information processing in the hippocampus, which is responsible for learning and short-term memory [4]. On the other hand, the excitatory neurons help share information to adjacent neurons, sending electrical signals called action potentials. This is possible because acetylcholine can bind to either a nicotinic receptor, which triggers an excitatory synaptic potential, or a muscarinic receptor, which triggers an inhibitory response [5]. For a person with AD, acetylcholine is in short supply [2].
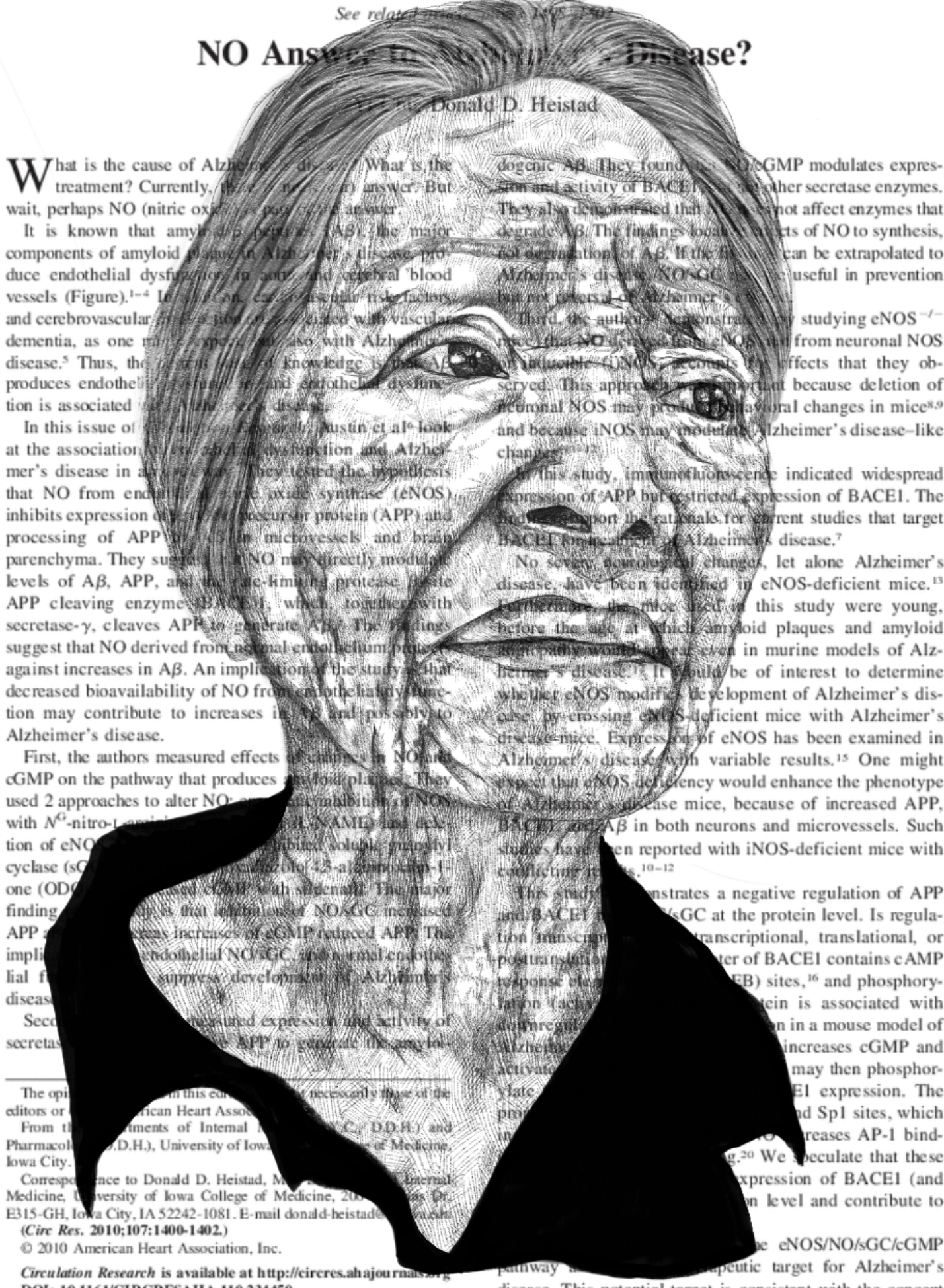
In the realm of AD research, the delicate balance of neurotransmitters comes under scrutiny, revealing crucial insights into the disease's progression. Among these neurotransmitters, acetylcholine takes center stage, with scientists uncovering a significant decrease in its release within AD patients [6]. This decline begins as an increase as acetylcholine is released by dying neurons in the early stages of the disease. Paradoxically, an excess of acetylcholine can lead to synaptic damage and contribute to the onset of AD. As neurons die, less acetylcholine is produced, and neighboring cellular functioning is damaged [6].
The body releases an enzyme called acetylcholinesterase (AChE) into the postsynaptic junctions of neurons [6]. AChE's role is to break down acetylcholine into citric acid and choline, effectively regulating its levels in the brain. In Alzheimer’s disease, AChE activity increases, which breaks down acetylcholine at a faster rate [6]. To exploit this mechanism, one common treatment approach for AD involves acetylcholinesterase inhibitors (AChEIs), which prevents acetylcholinesterase from breaking down acetylcholine, thus maintaining its activity in the brain for a longer amount of time [7].
Sometimes, age-dependent dementia causes much more than just memory damage. Many Alzheimer’s patients become short tempered and gain schizophrenic tendencies. The neurochemical alterations that cause AD are similar to the neurological deficits that occur in schizophrenic patients [8]. To combat that, the current standard of care is to prescribe antipsychotic medications [9]. However, these behaviors are associated with acetylcholine activity in the brain, and therefore may be able to be treated with AChEIs. For example, in 2016, researchers at the University of Exeter conducted a study to determine whether the usage of AChEIs in patients with mild to moderate AD could reduce the need for antipsychotic medication in the future [10]. The researchers wanted to explore the connection between acetylcholine, AD, and the various metabolites in cerebrospinal fluid, or CSF. This fluid runs through the ventricles and provides nutrients to the brain. This study had about 300 participants, all of whom had been diagnosed with AD and were, at the time, receiving antipsychotic medication for treatment. To study the effects of AChEIs, 50% of the participants were given AChEIs in addition to their current medications, whereas the other 50% of the participants received a placebo drug. The medication levels were measured using high-performance liquid chromatography (HPLC). This research method tracks cholesterol levels in the brain and allows us to see the movement of the drug through the brain. The researchers monitored the symptoms and assessed their need for any additional antipsychotic medication by performing a range of behavioral tests meant to diagnose psychosis, such as the Mini-Mental State Examination (MMSE) and the Montreal Cognitive Assessment (MoCA). The majority of the participants that received AChEIs showed a significant reduction in need for their additional medications. This study provides insight into potential targets to improve the quality of life of those with AD, as many of the antipsychotic medications had severe side effects in older adults, including cognitive impairment and increased risk of falls [10].
Cholinergic hypothesis
The cholinergic system, located in the subcortical region of the brain, regulates brain functions like attention, sleep, and arousal via acetylcholine receptors [11]. The cholinergic system is made up of nerve cells that use acetylcholine for action potentials [12]. When there is a reduction of cholinergic neurons, the acetylcholine activity also drops, which could contribute to the decreased acetylcholine levels in AD patients [13]. These cholinergic neurons are involved in physiological processes like attention, learning, memory, and response to stress [14]. Since ACHEIs help to slow the symptoms of AD, the degeneration of cholinergic neurons and the loss of ACh is a major contributor to cognitive decline in AD. Currently, one of the most widely recognized treatments for AD are cholinesterase inhibitors (ChEI), which target the cholinergic system [15]. Many AD patients with less hippocampal atrophy seem to respond better than the control. The first drug used to treat AD was a cholinesterase inhibitor, Tacrine, although this drug was soon taken off the market due to serious side effects. A more recent drug, Donepezil, is an acetylcholinesterase inhibitor, and resulted in significant improvements over 24 weeks with early-stage AD [7][15].
Scientists have used immunohistochemistry to document changing levels of cholinergic neurons in AD versus non-AD patients [16]. Immunohistochemistry is a technique that allows the visualization of target proteins located in cells or tissues. In one study, they stained vesicles containing acetylcholine to identify cholinergic neurons in both AD and non-AD patients [16]. The researchers found that patients with AD had a lower density of cholinergic neurons compared to the other group (non-AD patients), suggesting there may be a positive correlation between the decrease of cholinergic neurons and the decrease of acetylcholine. This study bridges the connection between the cellular and molecular changes in the brain [16].
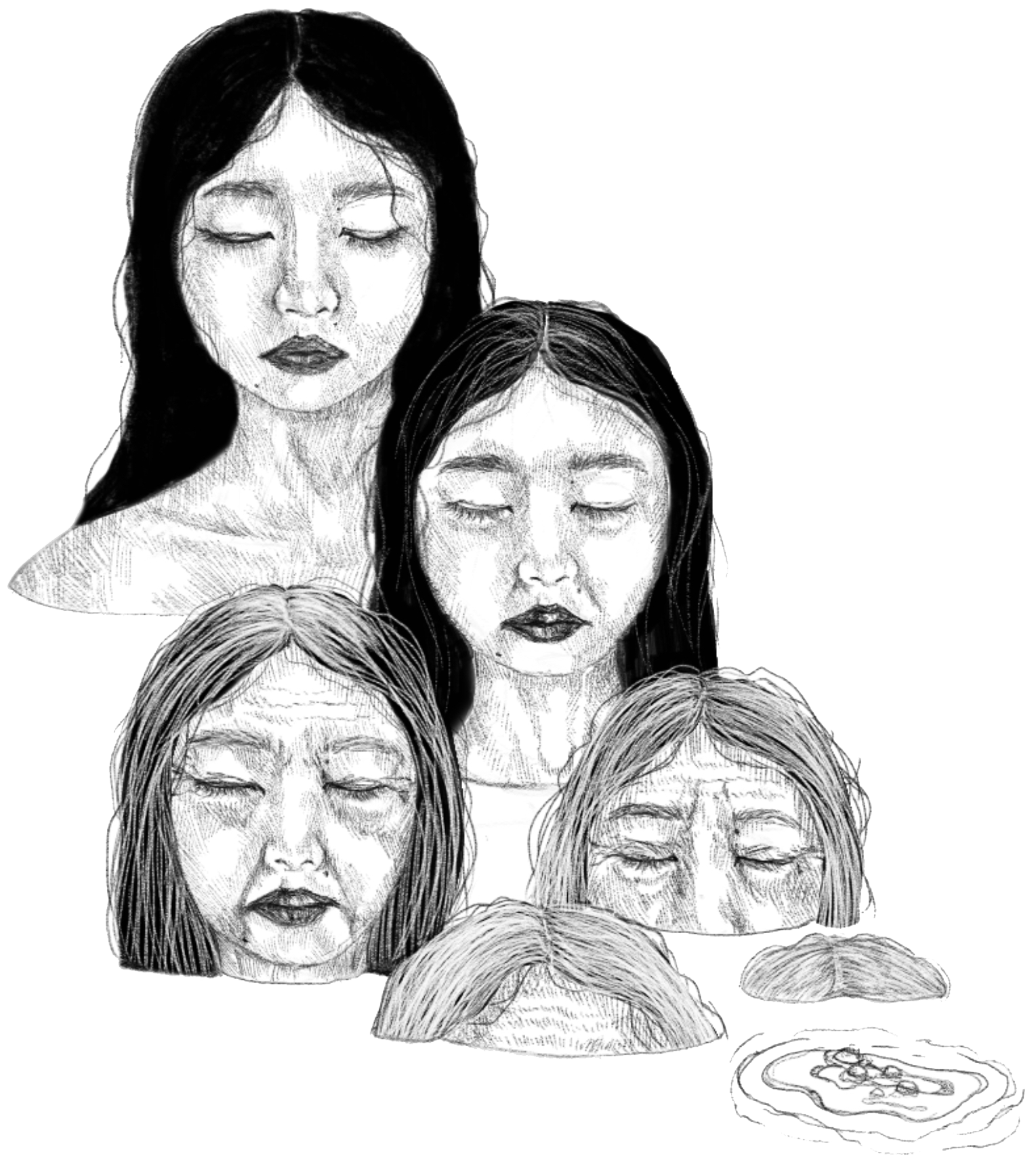
In another research study, 56 patients with mild to moderate AD were tested on their performance before and after ChEI treatment [17]. The scores from the MMSE showed that AD patients showed significant mental health recovery at their follow ups. Since this study tested the ChEI treatment on both patients with both mild and moderate AD, mild AD patients showed a higher average recovery compared to moderate patients [17]. However, it was concluded that there was insufficient evidence to determine the longevity of ChEI therapy [18]. The concept of treating AD patients with cholinesterase inhibitors is still somewhat new and its capacity to treat AD is still being researched today. It is important to note that cholinesterase inhibitors do help with symptomatic relief for AD patients, however, they do not directly target the pathology of the disease.
Current Treatments and Future Directions
Despite the ongoing quest for a definitive cure, the field of Alzheimer's disease research has witnessed the emergence of novel treatments aimed at alleviating the symptoms of this debilitating condition. Medications such as Donepezil provide a glimmer of hope for patients and their families [7] [15]. Additionally, the development of two new treatment therapies, Aducanemab and Lecanemab, has brought optimism to the field [19]. These drugs target the plaques associated with the onset of Alzheimer's disease, with the goal of clearing them from the brain. However, it is important to acknowledge that these treatments are not universally effective, and do not necessarily target any of the underlying mechanisms of disease, rather they target an indicator of the disease [19]. The journey toward finding a cure for AD is far from over, and it is crucial to rally the collective efforts of researchers, healthcare professionals, and society as a whole to continue the pursuit of better treatment options and ultimately find a viable cure. Through ongoing research and advancements in our understanding of this complex disease, we can strive to improve the lives of those affected by Alzheimer's and bring us closer to the day when this devastating illness is conquered.
References
- Cohen, A. D., Landau, S. M., Snitz, B. E., Klunk, W. E., Blennow, K., & Zetterberg, H. (2019). Fluid and PET biomarkers for amyloid pathology in Alzheimer's disease. Molecular and cellular neurosciences, 97, 3–17. https://doi.org/10.1016/j.mcn.2018.12.004
- Ferreira-Vieira, T. H., Guimaraes, I. M., Silva, F. R., & Ribeiro, F. M. (2016). Alzheimer's disease: Targeting the Cholinergic System. Current neuropharmacology, 14(1), 101–115. https://doi.org/10.2174/1570159x13666150716165726
- Sheffler ZM, Reddy V, Pillarisetty LS. Physiology, Neurotransmitters. [Updated 2022 May 8]. In: StatPearls [Internet]. Treasure Island (FL): StatPearls Publishing; https://www.ncbi.nlm.nih.gov/books/NBK539894/
- McQuiston A. R. (2014). Acetylcholine release and inhibitory interneuron activity in hippocampal CA1. Frontiers in synaptic neuroscience, 6, 20. https://doi.org/10.3389/fnsyn.2014.00020
- Picciotto, M. R., Higley, M. J., & Mineur, Y. S. (2012). Acetylcholine as a neuromodulator: cholinergic signaling shapes nervous system function and behavior. Neuron, 76(1), 116–129. https://doi.org/10.1016/j.neuron.2012.08.036
- Chen, Z. R., Huang, J. B., Yang, S. L., & Hong, F. F. (2022). Role of Cholinergic Signaling in Alzheimer's Disease. Molecules (Basel, Switzerland), 27(6), 1816. https://doi.org/10.3390/molecules27061816
- Alhazmi, H. A., & Albratty, M. (2022). An update on the novel and approved drugs for Alzheimer disease. Saudi pharmaceutical journal : SPJ : the official publication of the Saudi Pharmaceutical Society, 30(12), 1755–1764. https://doi.org/10.1016/j.jsps.2022.10.004
- Kasai, K., Shenton, M. E., Salisbury, D. F., Onitsuka, T., Toner, S. K., Yurgelun-Todd, D., Kikinis, R., Jolesz, F. A., & McCarley, R. W. (2003). Differences and similarities in insular and temporal pole MRI gray matter volume abnormalities in first-episode schizophrenia and affective psychosis. Archives of general psychiatry, 60(11), 1069–1077. https://doi.org/10.1001/archpsyc.60.11.1069
- Zuidema, S. U., Johansson, A., Selbaek, G., Murray, M., Burns, A., Ballard, C., & Koopmans, R. T. (2015). A consensus guideline for antipsychotic drug use for dementia in care homes. Bridging the gap between scientific evidence and clinical practice. International psychogeriatrics, 27(11), 1849–1859. https://doi.org/10.1017/S1041610215000745
- Cummings, J. L., Tong, G., & Ballard, C. (2019). Treatment Combinations for Alzheimer's Disease: Current and Future Pharmacotherapy Options. Journal of Alzheimer's disease : JAD, 67(3), 779–794. https://doi.org/10.3233/JAD-180766
- Li, X., Yu, B., Sun, Q., Zhang, Y., Ren, M., Zhang, X., Li, A., Yuan, J., Madisen, L., Luo, Q., Zeng, H., Gong, H., & Qiu, Z. (2018). Generation of a whole-brain atlas for the cholinergic system and mesoscopic projectome analysis of basal forebrain cholinergic neurons. Proceedings of the National Academy of Sciences of the United States of America, 115(2), 415–420. https://doi.org/10.1073/pnas.1703601115
- Jackson, C. (2011). Cholinergic System. Encyclopedia Of Clinical Neuropsychology, 562-564. doi: 10.1007/978-0-387-79948-3_1113
- Chen, Z. R., Huang, J. B., Yang, S. L., & Hong, F. F. (2022). Role of Cholinergic Signaling in Alzheimer's Disease. Molecules (Basel, Switzerland), 27(6), 1816. https://doi.org/10.3390/molecules27061816
- Du, X., Wang, X., & Geng, M. (2018). Alzheimer's disease hypothesis and related therapies. Translational neurodegeneration, 7, 2. https://doi.org/10.1186/s40035-018-0107-y
- Kumar A, Gupta V, Sharma S. Donepezil. [Updated 2021 Dec 22]. In: StatPearls [Internet]. Treasure Island (FL): StatPearls Publishing. https://www.ncbi.nlm.nih.gov/books/NBK513257/
- Hunter, J. M., Kwan, J., Malek-Ahmadi, M., Maarouf, C. L., Kokjohn, T. A., Belden, C., Sabbagh, M. N., Beach, T. G., & Roher, A. E. (2012). Morphological and pathological evolution of the brain microcirculation in aging and Alzheimer's disease. PloS one, 7(5), e36893. https://doi.org/10.1371/journal.pone.0036893
- Wakefield, S., Gardini, S., McGeown, W., Messa, G., Concari, L., Pellegrini, F.F., Ambrosecchia, M., Caffarra, P. and Venneri, A. (2012), P3-350: Cholinesterase inhibitor (ChEI) treatment benefits in mild compared to moderate Alzheimer's disease. Alzheimer's & Dementia, 8: P579-P579. https://doi.org/10.1016/j.jalz.2012.05.2172
- Hogan D. B. (2014). Long-term efficacy and toxicity of cholinesterase inhibitors in the treatment of Alzheimer disease. Canadian journal of psychiatry. Revue canadienne de psychiatrie, 59(12), 618–623. https://doi.org/10.1177/070674371405901202
- Verger, A., Yakushev, I., Albert, N. L., van Berckel, B., Brendel, M., Cecchin, D., Fernandez, P. A., Fraioli, F., Guedj, E., Morbelli, S., Tolboom, N., Traub-Weidinger, T., Van Weehaeghe, D., & Barthel, H. (2023). FDA approval of lecanemab: the real start of widespread amyloid PET use? - the EANM Neuroimaging Committee perspective. European journal of nuclear medicine and molecular imaging, 50(6), 1553–1555. https://doi.org/10.1007/s00259-023-06177-5