In April 2013, President Barack Obama announced an initiative to fund approximately one hundred million dollars of neuroscience research, called the Brain Research through Advancing Innovative Neurotechnologies Initiative (BRAIN). By encouraging and supporting the study of neurons, these funds are hoped to shed more light on human brain function. As wonderful as this is for neuroscience, BRAIN is forgetting about a crucial class of cells in the brain – glial cells.
Have you ever heard of glia? Most people know little about these brain cells, even though in some areas of the brain they outnumber neurons two to one [1].
Glial cells, or glia, are a family of non-neuronal brain cells that, unlike neurons, do not generate action potentials or have chemical synapses. Microglia are one of at least four different types of glial cells, each of which have distinct roles and abilities.
Microglia perform a variety of tasks that range from supporting synapse development to promoting neuron growth, and even apoptosis, or programmed cell death [2]. In fact, research suggests that microglia dysfunction is related to a slew of neurological disorders such as Alzheimer’s disease, Parkinson’s disease, depression, and schizophrenia [3].
How do microglia do so much? And how does our growing understanding contribute to the pathology of the disorders mentioned above? Research on microglia has increased dramatically in the last twenty years with the simple goal of understanding what role microglia have in normal brain functioning.
History of Microglia
Scientists first discovered glial cells in the late 19th century, thanks to the groundbreaking work of Rudolf Virchow. Microglia, however, weren’t specifically identified until the early 20th century by neuroscientist Pio del Rio-Hortega.
Already there existed an understanding that the discovered glial cells were unlike typical neurons. Microglia were not specifically involved in basic neuronal signaling; rather, they possessed an ability to change shape depending on their environment [4].
Unfortunately, research on microglia seemed to go on hiatus shortly after their discovery. Perhaps because neurons were easier to study, it wasn’t until the late 20th century when new techniques were developed that allowed neuroscientists to answer specific questions about microglia and their dynamic behavior.
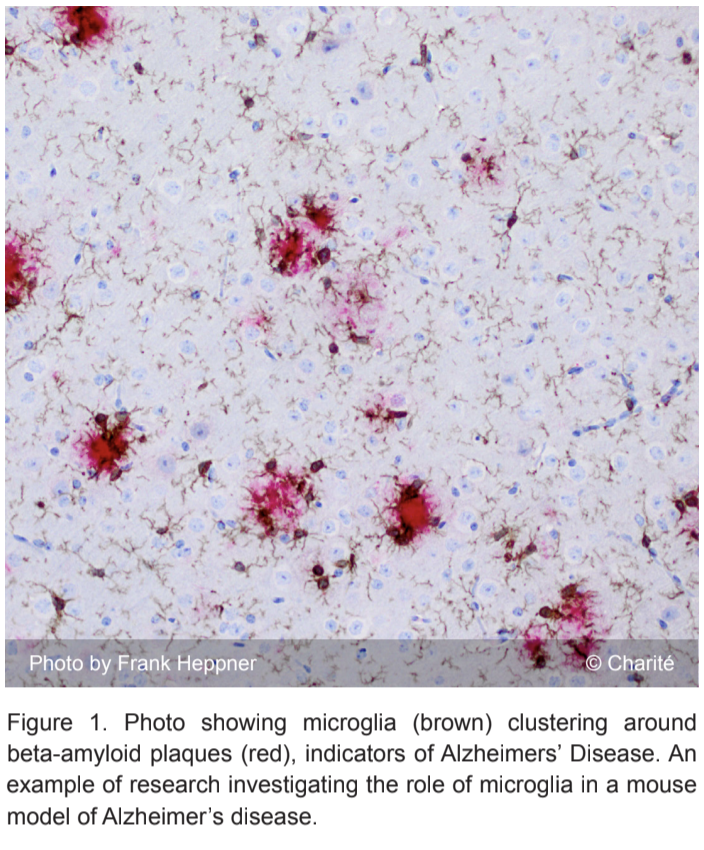
Current Understanding
It is thought that microglia are akin to macrophages, one of the key cells in immune response and healing. Similar to macrophages, microglia are derived from bone marrow and are indeed involved in immune responses in the brain such as phagocytosis, or the engulfing of damaged cells and other extracellular material [5].
Microglia are the resident immune cells in the central nervous system (CNS). They defend the brain from any type of inflammatory stimulus such as pathogens, blood loss, disease, and more. They have the ability to move throughout the brain and help form the supporting structure of the CNS [6]. Microglia are also very sensitive to their environment and alter their physiology according to changes in brain chemistry and homeostasis. This will be further explored in later paragraphs.
While they were once thought to be inactive for brief moments, microglia are never idle, as they constantly scan the brain. It is estimated that microglia are able to scan nearly the entire brain in just a few hours, searching for interlopers [7].
When triggered by stimuli such as CNS injury or disease, microglia rapidly undergo activation through complex pathways before changing structure to better face the stimulus [6].
In addition, recent research indicates that microglia seem to be involved in early brain development. Neuroscientists have revealed evidence that microglia contribute to synaptic development and pruning in the brain’s early stages of growth – an important role to say the least [8].
So how do microglia accomplish such a variety of tasks? It has been known for decades that microglia are capable of altering their morphology, or shape, in order to change their function, making them extremely diverse [6]. This ability to switch between phenotypes has prompted scientists ask what regulates these changes and what happens when the mechanisms involved are disrupted.
Researchers discovered that there are actually two main morphologies that microglia can express: ramified or amoeboid.
Ramified Microglia
Ramified microglia, or “resting microglia,” are present in a mature and healthy brain. This type of microglia was once thought to simply rest in the CNS, awaiting activation; however, publications are rapidly emerging, revealing that ramified microglia are active participants not only in maintaining a healthy brain, but also in supporting a developing one.
Moving about the brain’s tissues, resting microglia do not simply “rest.” They utilize chemotaxis to patrol for potential targets such as pathogens or cells that have undergone apoptosis [9]. They have short, protruding processes that move around the cell body as the microglia move. These serve to increases the surface area of the microglial cell so that it can be more efficient in scanning the CNS [6].
The ramified processes can be extended or retracted in mere minutes [10]. These extensions can even reach out and make contact with other cells such as astrocytes, pre-synaptic neurons, or post-synaptic neurons. This suggests that microglia might posses the ability to regulate signal transmission, synapse development, and even plasticity – the brain’s ability to change due to experience.
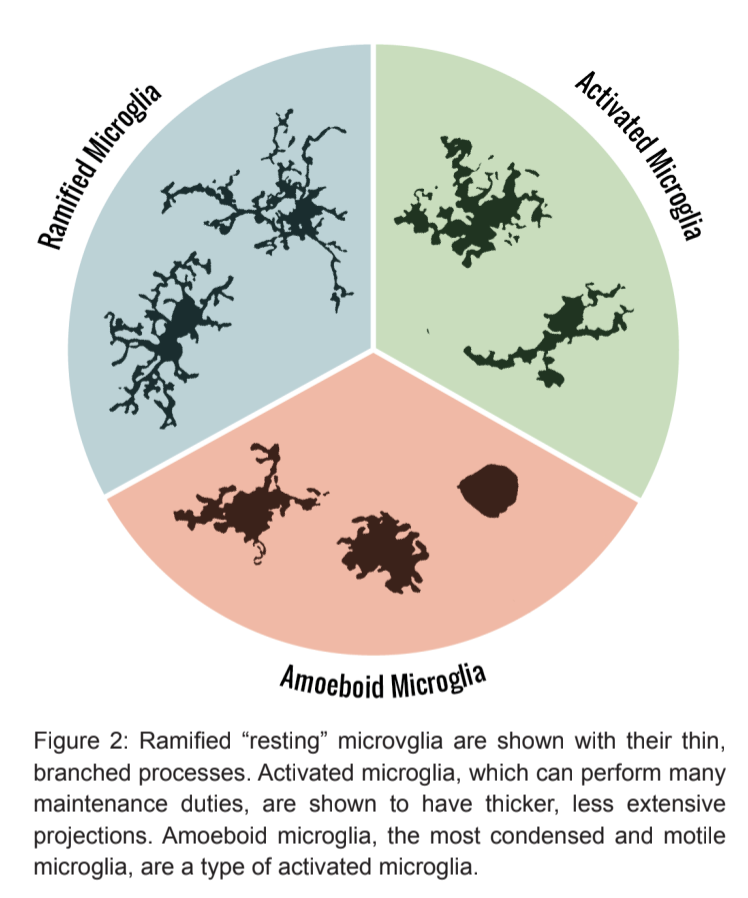
Ramified microglia can perform phagocytosis even in the absence of their normal inflammatory stimuli8. It all depends on where in the brain these microglia are located. If, for example, they are in the hippocampus, which is a structure highly involved in memory formation and organization, resting microglia might adopt this function to help prune and rearrange synapses [11].
The science behind microglia in the healthy adult brain is still relatively new; however, most studies hint at the idea that microglia are not solely involved in responding to an injured brain. New research has just started to address how a given location in the brain influences ramified microglia behavior, as well as the extent to which these microglia contribute to normal brain development.
Amoeboid ("Activated") Microglia
Microglial activation occurs when a ramified microglial cell comes into contact with anything that disrupts homeostasis within the brain. Once activated, the microglial cell undergoes changes in gene expression and rapidly alters its shape to better attack the disrupting stimuli [12].
Activated microglia adopt the amoeboid morphology – these cells are able to move around, usually towards their target, and they retract their processes, becoming more spherical [6]. These blob-shaped microglia are still very environment-specific; therefore, activated microglia can appear and behave differently in one area of the brain versus another.
Each specific response, or activation state, also depends on the stimuli, since amoeboid microglia function changes depending on what it’s targeting. In certain cases, activated microglia can be stimulated to become alternatively activated, or neuroprotective [13].
Alternatively, activated microglia can opt to release neuronal growth factors that serve to aid damaged neurons in regeneration. They can also prepare to engulf cellular debris or release molecules such as cytokines that are involved in an anti-inflammatory response. In addition, the microglial cell can phagocytize anything it needs to remove from the surrounding brain tissue to help the brain return to homeostasis [14].
Microglia can also be “fully” or “over” activated, in which they actually become neurotoxic to some extent. They are then involved in a pro-inflammatory response, releasing nitric oxide, cytokines, and reactive oxygen species that physically cause neuronal damage, or neurodegeneration [15].
When properly regulated, this sort of response can be beneficial to the brain. Constant or chronic activation of microglia, however, can be potentially injurious to the surrounding brain cells, leading to neurodysfunction.
Essentially, activated microglia are effective in supporting and helping regenerate neurons, as well as removing pathogens and inflammatory stimuli through phagocytosis and the like. Microglia activation can also occur in a way that is actually detrimental to brain health and function.
Neurodegenerative Disease
The complete mechanisms behind the activation pathways of microglia are not currently understood, but we do know that an aging brain’s microglia tend to behave more abnormally. In younger brains, microglia seem to prefer activation through the neuroprotective route, while in aging brains, microglia are more prone to over-activation and thus neurodegeneration [16].
Questions remain regarding the changes in microglial response that could lead to neurodegeneration. Is it due to microglial dysfunction? How much of a role does the aging brain play? When one considers the fact that Alzheimer and Parkinson’s diseases are neurodegenerative and partially brought upon by old age, it is fair to postulate that dysfunctional microglia may contribute to the degeneration.
This opens up a whole new range of possible therapies for neurodegenerative diseases. Could microglia and regulation of activation be an answer to mitigating symptoms such as loss of brain cells or impaired brain function? This is one of many questions left to answer regarding microglia.
Conclusion
Microglia, the cells once thought to passively guard the brain, are now being shown to have expansive roles in the CNS. Although they are slightly less mysterious than they were even 20 years ago, microglia still remind us that we have much left to learn about the brain. Only further questioning and research can uncover all that this dynamic cell can do. Until then, neuroscientists can only continue to unravel the tantalizing mystery that is microglia.
References
- Parkhurst, C.N., Yang, G., Ninan, I., Savas, J.N., Yates III, J.R., Lafaille, J.J., Hempstead B.L., Littman, D.R., Gan, W. (2013). “Microglia Promote Learning-Development Synapse Formation through Brain-Derived Neutrophic Factor”. Cell 155 (7): 1596-1609. doi: 10.1016/j.cell.2013.11.030.
- Rogers, J., Mastroeni, D., Leonard, B., Joyce, J., Grover, A., (2007). “Neuroinflammation in Alzheimer’s disease and Parkinson’s disease: are microglia pathogenic in either disorder?”. International Review of Neurobiology 82: 235-246. doi: 10.1016/S0074-7742(07)82012-5
- Tambuyzer, Bart R., Peter Ponsaerts, and Etienne J. Nouwen. "Microglia: gatekeepers of central nervous system immunology." Journal of leukocyte biology 85.3 (2009): 352-370.Dfa
- Soulet, D., Rivest, S., (2008). “Bone-marrow-derived microglia: myth or reality?” Current Opinion in Pharmacology 8 (4): 508-518. doi: 10.1016/j.coph.2008.04.002
- Kettenmann, H., Hanisch, U., Noda, M., Verhratsky, A., (2011). “Physiology of Microglia”. Physiological Reviews 91: 461-553. doi:10.1152/physrev.00011.2010
- Hefendehl, J. K., Neher, J. J., Sühs, R. B., Kohsaka, S., Skodras, A. and Jucker, M. (2014), Homeostatic and injury-induced microglia behavior in the aging brain. Aging Cell, 13: 60–69. doi: 10.1111/acel.12149
- Kettenmann, H., Hanisch, U., Noda, M., Verhratsky, A., (2011). “Physiology of Microglia”. Physiological Reviews 91: 461-553. doi:10.1152/physrev.00011.2010
- Tremblay, M., Stevens, B., Sierra, A., Wake, H., Bessis, A., Nummerjahn, A. (2011). “The Role of Microglia in the Healthy Brain.” The Journal of Neuroscience 31 (45): 16064-16069. doi: 10.1523/JNEUROSCI.4158-11.2011
- Kettenmann, H., Hanisch, U., Noda, M., Verhratsky, A., (2011). “Physiology of Microglia”. Physiological Reviews 91: 461-553. doi:10.1152/physrev.00011.2010
- Liu, G. J., Nagarajah, R., Banati, R. B. and Bennett, M. R. (2009), Glutamate induces directed chemotaxis of microglia. European Journal of Neuroscience, 29: 1108–1118. doi: 10.1111/j.1460-9568.2009.06659.x
- Kettenmann, H., Hanisch, U., Noda, M., Verhratsky, A., (2011). “Physiology of Microglia”. Physiological Reviews 91: 461-553. doi:10.1152/physrev.00011.2010
- Orr, A., Orr, A., Li, X., Gross R., Traynelis, S., (2009). “Adenosine A2A receptor mediated microglial process retraction”. Nature Neuroscience 12: 872-878. doi: 10.1038/nn.2341
- Tremblay, M., Stevens, B., Sierra, A., Wake, H., Bessis, A., Nummerjahn, A. (2011). “The Role of Microglia in the Healthy Brain.” The Journal of Neuroscience 31 (45): 16064-16069. doi: 10.1523/JNEUROSCI.4158-11.2011
- Rock, R. Bryan, et al. "Role of microglia in central nervous system infections." Clinical Microbiology Reviews 17.4 (2004): 942-964.
- Thomas, David M., Dina M. Francescutti-Verbeem, and Donald M. Kuhn. "Gene expression profile of activated microglia under conditions associated with dopamine neuronal damage." The FASEB journal 20.3 (2006): 515-517.
- Kettenmann, H., Hanisch, U., Noda, M., Verhratsky, A., (2011). “Physiology of Microglia”. Physiological Reviews 91: 461-553. doi:10.1152/physrev.00011.2010
- Ekdahl, C., (2012). “Microglial Activation – Tuning and Pruning Adult Neurogenesis”. Frontiers in Pharmacology. doi: 10.3389/fphar.2012.00041
- Ibid.
- Block, M., Zecca, L., Hong, J., (2007). “Microglia-mediated neurotoxicity: uncovering the molecular mechanisms”. Nature Reviews Neuroscience (8): 57-69. doi: 10.1038/nrn2038.
- Norden, D., Godbout, J., (2013). “Microglia of the Aged Brain: Primed to be Activated and Resistant to Regulation”. 39 (1): 19-34. doi: 10.1111/j.1365-2990.2013.01306.x