What is the Spiny Mouse?
How amazing would it be to possess the ability to regenerate lost body parts? It would be effortless to easily produce new limbs, organs, or even parts of your brain after loss of function. The ability to automatically regenerate parts of your body would spare many patients from prolonged pain, time-consuming rehabilitation, and trips to the hospital, which are all problems individuals currently face when it comes to healthcare for injuries and amputations. Although we are far from possessing similar regenerative powers, regeneration at the cellular level is slowly becoming within reach.
Within the broad range of injuries that humanity commonly encounters, a devastating trauma is found in the spinal cord. Spinal cord injury is a disorder resulting from trauma that can greatly disrupt an individual’s bodily functions. The most desirable physical goal in spinal cord injury cases is for the patient to fully recover the ability to stand and move independently. However, there are also welfare issues that must be solved - for most spinal cord injury patients, bladder, bowel, and sexual function are usually ranked as the most important factors impacting their quality of life [1]. Treatments for humans remain largely palliative, meaning that we can only mitigate a patient’s suffering without actually curing the problem. In order to help patients more easily, or completely, recover from spinal cord injuries, researchers are looking at how the tissue itself can regenerate, rather than heal. Healing is one of the tissue’s natural responses to injury, but during the process, scars form that affect the ability of the tissue to be fully functional again [2]. Regeneration, however, results in complete restoration. Neuroscientists are gaining more and more knowledge about how certain spinal cell tissue components recover after spinal cord injury.
Researchers commonly model ailments using animals that have a similar genetic makeup to humans [3]. This allows a way for research to be done without using human subjects. One model that is utilized often is the common laboratory mouse, also known as Mus Musculus. Not only can genes easily be located in the genome sequence of Mus Musculus, but they can also be easily manipulated to determine the function of those genes [3]. Although Mus Musculus is a good animal model for some disease studies, this genus does not have the ability to regenerate tissue, making it a poor model for understanding how tissue regeneration functions [3]. Instead, neuroscientists are studying another genus of mice that has the potential to fully recover mobility and organ functionality after damage to the spine.
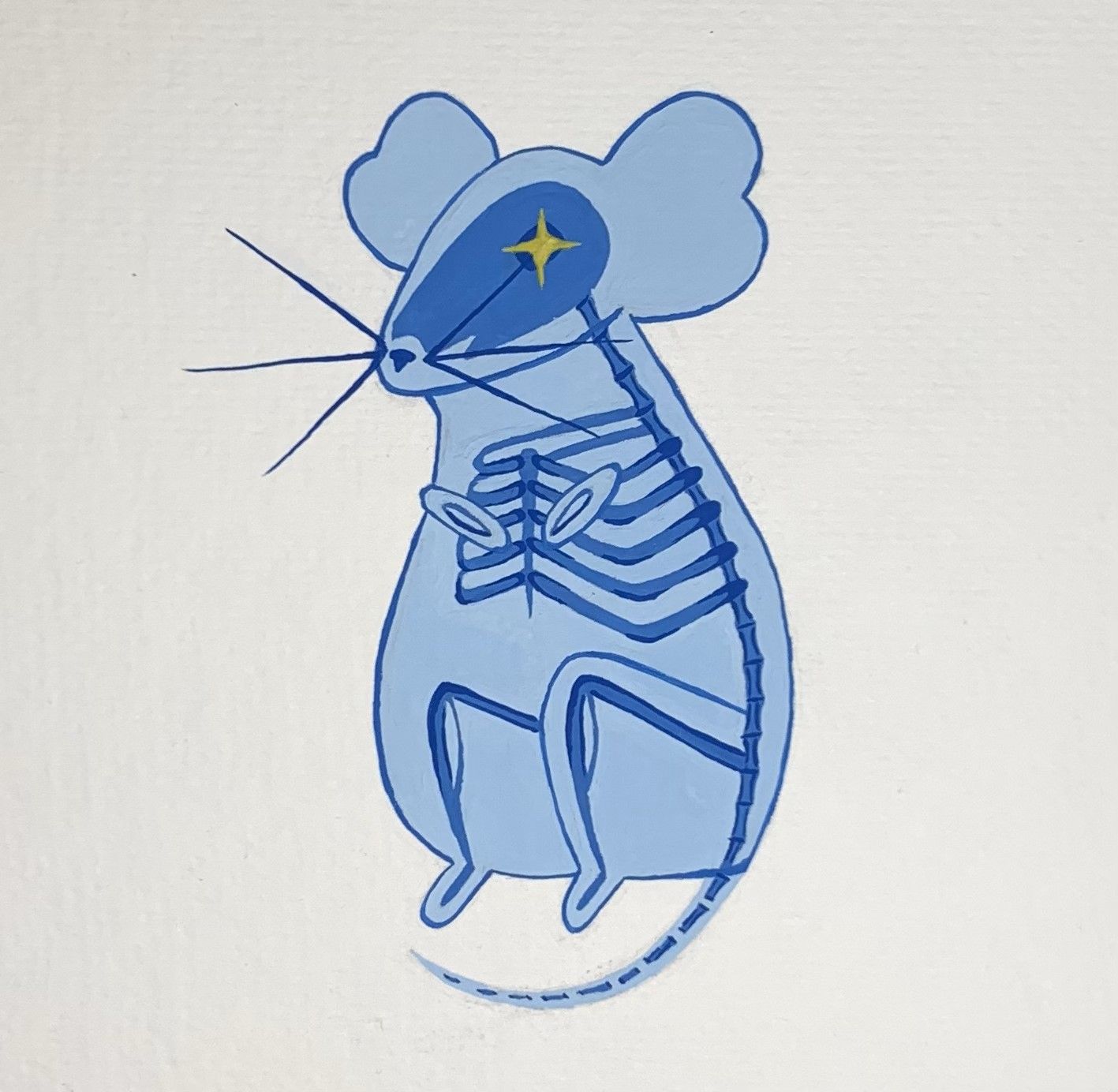
Acomys, also known as the spiny mouse, is a genus of mouse that possesses many unique abilities and features not seen in Mus Musculus and other rodents [4]. It is found mostly in Africa, the Middle East, and southern Asia. Currently, scientists recognize eighteen different spiny mouse species that possess various distinctive features at birth, such as fur, open eyes, and unfolded ears. Spiny mouse pups are also born with mostly developed livers, kidneys, lungs, and brains, allowing them to mature faster than typical rodents who begin maturing these organs after birth [4]. Perhaps the most curious feature of spiny mice is their ability to regenerate damaged complex tissues [5]. The CNS is the body’s processing center and consists of the brain and spinal cord. It was previously thought that mammals could not fully recover from serious spinal cord or other CNS injuries since the axons of most nerve cells in the adult CNS cannot spontaneously regenerate after injury [6] - that is, until the spiny mouse was discovered.
The spiny mouse is able to regrow skeletal muscle, fully heal skin wounds without scarring, and regenerate cells in their central nervous system (CNS) to full functionality after injury [6]. In an experiment studying their response to spinal cord injuries, researchers severed the spinal cords at the T8 (thoracic) vertebrae of fourteen spiny mice and fourteen Mus Musculus [7]. Remarkably, eight spiny mice regained complete mobility, going from half-paralyzed, or paraplegic, to normal mobility in eight weeks, while Mus Musculus mobility remained dysfunctional [7]. Spinal cord injury lesions cause failure of CNS nerve axon regrowth in adult mammals, so the fact that spiny mice can naturally overcome this lack of regeneration is incredible [8]. Due to this ability, spiny mice have recently emerged as a prime mammalian model for CNS regeneration research. Spiny mouse research is ongoing as scientists strive to understand the mechanisms behind their regeneration.
Spiny Mouse Regenerative Research
In order to determine the extent to which spiny mice can regenerate their CNS cells, neuroscientists visualize cells in spinal cord cross-sections through a process called immunohistochemistry (IHC) staining [9]. IHC staining uses antibodies specifically targeted for certain antigens in tissue samples from model organisms in order to locate and visualize cell components [9]. Antibodies are immune proteins that recognize foreign agents called antigens. This identification is done through a primary and secondary stain. In a primary stain, scientists soak the tissue cross-sections from spiny mice with primary antibodies from a rabbit [7]. Since a rabbit’s antibodies are used, proteins in the spiny mouse tissue are detected as foreign antigens, causing the rabbit antibodies to bind to them as an immune response. A secondary stain is then completed to colorize the bound proteins using an antibody called Alexa Fluor. The secondary antibodies must also be from a different animal than the primary antibodies in order to bind. In the case of rabbit primary antibodies, scientists may use Alexa Fluor donkey antibodies in order to tag the previously bound proteins with fluorescence. This process makes the spiny mouse proteins visible under a microscope that fluoresces specific wavelengths in colors such as red, green, or blue. Scientists can then look at the structures of the proteins in question, count them, and compare the prevalence of that certain cell component between different animal tissues or different tissue treatments [7].
When using the IHC method, scientists usually stain for specific CNS cells and proteins in both spiny mice and common lab mice tissue samples that they believe to be important in the process of regeneration. By comparing spiny mice and Mus Musculus samples, scientists can pinpoint which proteins and CNS cells may be responsible for the spiny mouse’s heightened regeneration [7]. For instance, the primary antibody collagen IV is used to mark and visualize tissue scarring, which shows up under a microscope as large colored gashes where there is scar tissue [10]. Researchers found that there is reduced prevalence of these gashes in spiny mouse tissue compared with Mus Musculus tissue following injury [7].
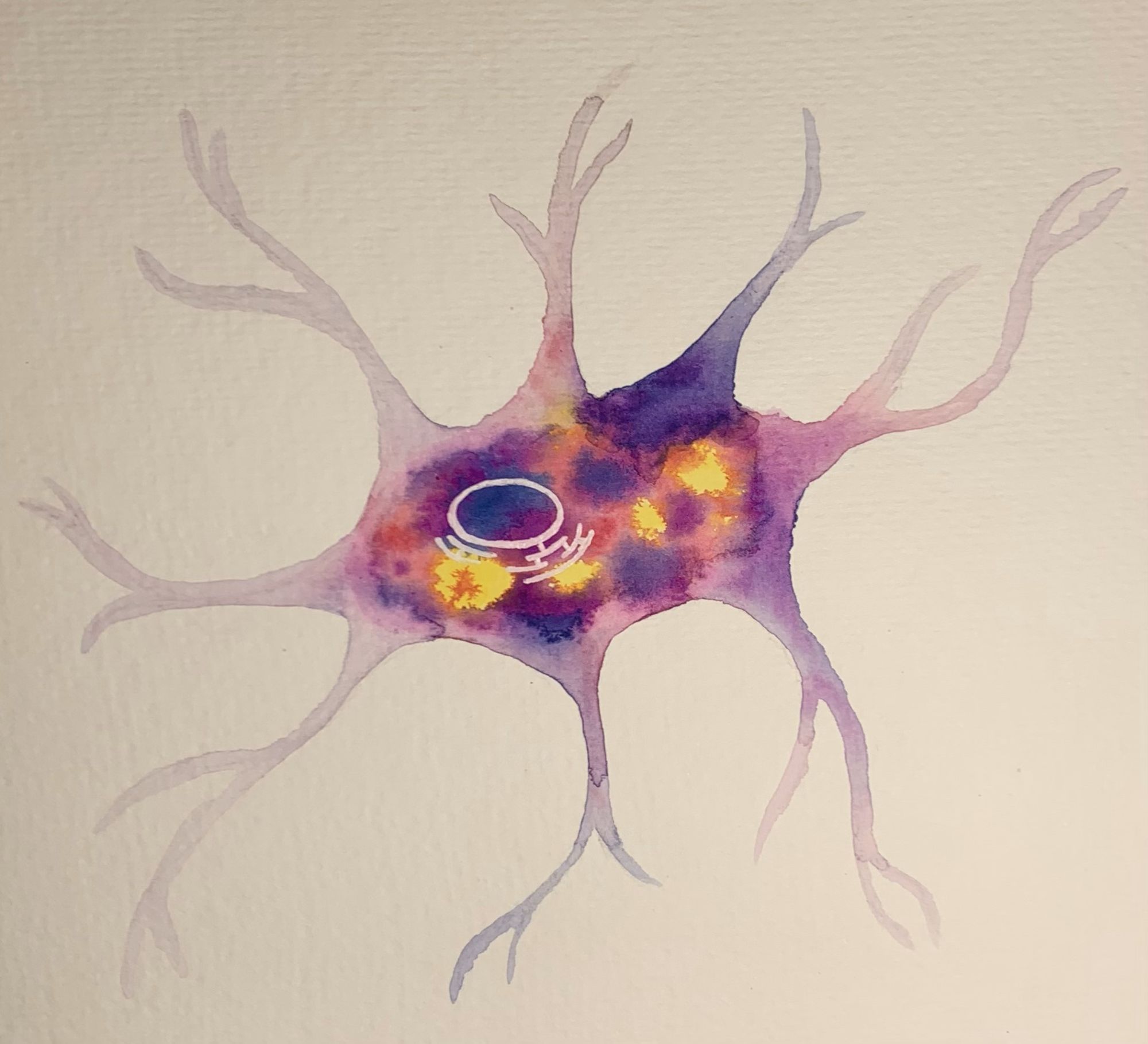
Other antibodies spiny mouse researchers use are glial fibrillary acidic protein (GFAP) and ionized calcium binding adaptor molecule 1 (IBA1) [11]. GFAP makes astrocyte glial cells visible under a microscope, while IBA1 marks and visualizes microglial cells [11]. Broadly, glia are cells in the nervous system that support by helping them carry out their many functions, such as defining contact between synapses and preserving their signaling abilities. Astrocyte glial cells are a specific type of glia, compose the majority of the CNS in mammals, so studying potential structural differences of astrocyte glial cells between the spiny mouse and Mus Musculus could give more insight into spiny mouse regeneration [12]. Microglial cells are the first responders to a CNS injury and are believed to promote regeneration, making them a prime CNS cell to be studied. Through these specific IHC techniques, scientists found that Acomys spinal cord cross-sections following injury will exhibit less tissue scarring and greater microglial cell numbers than Mus Musculus if the spiny mouse can heal its cells more efficiently [7].
Researchers tested these hypotheses using IHC staining on spinal cord sections that were harvested four weeks following spinal cord injury [10]. As predicted, microscope imaging of these tissues indicated less spinal scarring in spiny mice compared to Mus Musculus. These findings indicate that the spiny mouse is able to fully repair CNS injuries faster than both laboratory mice and humans by regenerating tissue cells more efficiently. GFAP and IBA1 stains also showed a difference in the appearance of astrocytes and microglia in spiny mice, as cells seemed to be physically less thick than in Mus Musculus. Scientists are not yet confident in the reasons for why these results are seen, but through further research, more information will be revealed to help make assertive conclusions [10].
IHC is not the only set of methods that can be used in spiny mouse regeneration experimentation. Researchers have found that the spiny mouse exhibits a more rapid recovery of bladder function compared to Mus Musculus by using manual bladder expression twice a day after spinal cord injury [13]. This is a reference to neurogenic bladder, a symptom of spinal cord injury which causes a patient to lose control of their bladder. The fact that the spiny mouse was able to restore its bladder function within forty-eight hours of spinal cord injury, compared to more than two weeks in a regular mouse, further supports the idea that the spiny mouse is able to regenerate and heal its CNS more efficiently compared to Mus Musculus. Researchers also found that the spiny mouse upregulates more genes associated with neurogenesis following injury [13]. This means that spiny mouse cells increase their response to a specific signal to form new neurons and nerve tissue. However, more research is necessary to understand the signal and cell mechanisms involved in the formation of new tissue to find out why and how spiny mouse cells are more responsive. These studies and findings are a promising start to explaining why the spiny mouse is able to heal a spinal cord injury more successfully than other mammals.
Future Directions
Further research is required to better understand the mechanisms behind the spiny mouse’s incredible regenerative abilities. While scientists value the spiny mouse as a model for regenerative research, focus on mechanisms that reduce scarring and increase neurogenesis in future studies is crucial for fully understanding CNS regeneration [13]. For instance, identifying whether or not the spiny mouse’s immune and fibrotic responses result in improved axon regrowth across an injured area would be a prime topic of experimentation [14].
More specific biological factors found to be present in spiny mouse regeneration are also of interest for future experimentation. The enzyme beta-3gnt7, for example, is one of those components [7]. This enzyme is a crucial driver of various biological reactions and processes in the connective tissues between cells, also known as the extracellular matrix [15]. The extracellular matrix may hold significant information to axon regeneration [16]. Researchers would like to see if the overexpression of beta-3gnt7 in a non-regenerative competent species, such as the common laboratory mouse, results in regrowth of cell axons. Studies on specific enzymes such as beta-3gnt7 or other certain proteins present in CNS cells would help us further analyze the role of certain pathways in biosynthesis that may promote regeneration [7].
Applications in Human Medicine
Mouse studies are translational to humans because scientists can mimic in mice the effect of certain DNA alterations that may occur in human diseases. Disease can be instigated by induction of the illness, allowing researchers to easily study immune responses [17]. If neuroscientists can discover more about the spiny mouse’s regenerative abilities, their knowledge could discover further information behind CNS recovery and help healthcare professionals better treat humans with spinal cord injury.
Research highlights the importance of investigating CNS regeneration outside traditional model organisms, such as the lab mouse, and instead points us towards studying mammals that possess an increased capacity for CNS regeneration [18]. The spinal regeneration abilities of the spine mouse following trauma sets it apart from any other animal as a unique model to investigate mammalian CNS regeneration. New knowledge from spiny mouse research may teach us what is required for cellular regrowth in other organisms and can aid in the goal of creating novel treatments, or perhaps even a cure, for various CNS injuries in humans.
Unfortunately, it seems clear that humans cannot quite mimic the regenerative superpowers of various other animals. Nonetheless, once scientists discover the mechanisms behind regeneration in model organisms like the spiny mouse, then our understanding of healing severe injuries in which cells are unable to regrow by themselves will be greatly strengthened. With this knowledge, researchers and healthcare professionals will be one step closer to developing novel therapies beyond palliative care that can effectively treat and heal spinal cord injury in humans.
References
- Anderson K. D. (2004). Targeting recovery: priorities of the spinal cord-injured population. Journal of neurotrauma, 21(10), 1371–1383. https://doi.org/10.1089/neu.2004.21.1371
- Rabiller, L., Robert, V., Arlat, A., Labit, E., Ousset, M., Salon, M., Coste, A., Da Costa-Fernandes, L., Monsarrat, P., Ségui, B., André, M., Guissard, C., Renoud, M. L., Silva, M., Mithieux, G., Raymond-Letron, I., Pénicaud, L., Lorsignol, A., Casteilla, L., Dromard Berthézène, C., … Cousin, B. (2021). Driving regeneration, instead of healing, in adult mammals: the decisive role of resident macrophages through efferocytosis. NPJ Regenerative medicine, 6(1), 41. https://doi.org/10.1038/s41536-021-00151-1
- Yue, F., Cheng, Y., Breschi, A., Vierstra, J., Wu, W., Ryba, T., Sandstrom, R., Ma, Z., Davis, C., Pope, B. D., Shen, Y., Pervouchine, D. D., Djebali, S., Thurman, R. E., Kaul, R., Rynes, E., Kirilusha, A., Marinov, G. K., Williams, B. A., Trout, D., … Mouse ENCODE Consortium (2014). A comparative encyclopedia of DNA elements in the mouse genome. Nature, 515(7527), 355–364. https://doi.org/10.1038/nature13992
- Haughton, C. L., Gawriluk, T. R., & Seifert, A. W. (2016). The Biology and Husbandry of the African Spiny Mouse (Acomys cahirinus) and the Research Uses of a Laboratory Colony. Journal of the American Association for Laboratory Animal Science : JAALAS, 55(1), 9–17.
- Imran, S. A. M., M Hamizul, M. H. A., Khairul Bariah, A. A. N., Wan Kamarul Zaman, W. S., & Nordin, F. (2022). Regenerative Medicine Therapy in Malaysia: An Update. Frontiers in bioengineering and biotechnology, 10, 789644. https://doi.org/10.3389/fbioe.2022.789644
- Canty, A. J., Huang, L., Jackson, J. S., Little, G. E., Knott, G., Maco, B., & De Paola, V. (2013). In-vivo single neuron axotomy triggers axon regeneration to restore synaptic density in specific cortical circuits. Nature communications, 4, 2038. https://doi.org/10.1038/ncomms3038
- Nogueira-Rodrigues, J., Leite, S. C., Pinto-Costa, R., Sousa, S. C., Luz, L. L., Sintra, M. A., Oliveira, R., Monteiro, A. C., Pinheiro, G. G., Vitorino, M., Silva, J. A., Simão, S., Fernandes, V. E., Provazník, J., Benes, V., Cruz, C. D., Safronov, B. V., Magalhães, A., Reis, C. A., Vieira, J., … Sousa, M. M. (2022). Rewired glycosylation activity promotes scarless regeneration and functional recovery in spiny mice after complete spinal cord transection. Developmental cell, 57(4), 440–450.e7. https://doi.org/10.1016/j.devcel.2021.12.008
- Anderson, M. A., O'Shea, T. M., Burda, J. E., Ao, Y., Barlatey, S. L., Bernstein, A. M., Kim, J. H., James, N. D., Rogers, A., Kato, B., Wollenberg, A. L., Kawaguchi, R., Coppola, G., Wang, C., Deming, T. J., He, Z., Courtine, G., & Sofroniew, M. V. (2018). Required growth facilitators propel axon regeneration across complete spinal cord injury. Nature, 561(7723), 396–400. https://doi.org/10.1038/s41586-018-0467-6
- Magaki, S., Hojat, S. A., Wei, B., So, A., & Yong, W. H. (2019). An Introduction to the Performance of Immunohistochemistry. Methods in molecular biology (Clifton, N.J.), 1897, 289–298. https://doi.org/10.1007/978-1-4939-8935-5_25
- Streeter, K. A., Sunshine, M. D., Brant, J. O., Sandoval, A. G. W., Maden, M., & Fuller, D. D. (2020). Molecular and histologic outcomes following spinal cord injury in spiny mice, Acomys cahirinus. The Journal of comparative neurology, 528(9), 1535–1547. https://doi.org/10.1002/cne.24836
- Norden, D. M., Trojanowski, P. J., Villanueva, E., Navarro, E., & Godbout, J. P. (2016). Sequential activation of microglia and astrocyte cytokine expression precedes increased Iba-1 or GFAP immunoreactivity following systemic immune challenge. Glia, 64(2), 300–316. https://doi.org/10.1002/glia.22930
- Sofroniew, M. V., & Vinters, H. V. (2010). Astrocytes: biology and pathology. Acta neuropathologica, 119(1), 7–35. https://doi.org/10.1007/s00401-009-0619-8
- Gaire, J., Varholick, J. A., Rana, S., Sunshine, M. D., Doré, S., Barbazuk, W. B., Fuller, D. D., Maden, M., & Simmons, C. S. (2021). Spiny mouse (Acomys): an emerging research organism for regenerative medicine with applications beyond the skin. NPJ Regenerative medicine, 6(1), 1. https://doi.org/10.1038/s41536-020-00111-1
- Maden, M., & Varholick, J. A. (2020). Model systems for regeneration: the spiny mouse, Acomys cahirinus. Development (Cambridge, England), 147(4), dev167718. https://doi.org/10.1242/dev.167718
- Takeda-Uchimura, Y., Nishitsuji, K., Ikezaki, M., Akama, T. O., Ihara, Y., Allain, F., & Uchimura, K. (2022). Beta3Gn-T7 Is a Keratan Sulfate β1,3 N-Acetylglucosaminyltransferase in the Adult Brain. Frontiers in neuroanatomy, 16, 813841. https://doi.org/10.3389/fnana.2022.813841
- Yue B. (2014). Biology of the extracellular matrix: an overview. Journal of glaucoma, 23(8 Suppl 1), S20–S23. https://doi.org/10.1097/IJG.0000000000000108
- .Sellers RS. Translating Mouse Models: Immune Variation and Efficacy Testing. Toxicologic Pathology. 2017;45(1):134-145. doi:10.1177/0192623316675767
- Ghosh, S., & Hui, S. P. (2016). Regeneration of Zebrafish CNS: Adult Neurogenesis. Neural plasticity, 2016, 5815439. https://doi.org/10.1155/2016/5815439